It was the
good fortune of the Cambridge school to produce, in the Victorian
period, some of the greatest physicists of the century.
good fortune of the Cambridge school to produce, in the Victorian
period, some of the greatest physicists of the century.
Cambridge History of English Literature - 1908 - v14
.
.
.
The king now loaded one of the carbines I had given him with his
own hands, and giving it full cock to a page, told him to go out and shoot
a man in the outer court; which was no sooner accomplished than the little
urchin returned to announce his success with a look of glee such as one would
see in the face of a boy who had robbed a bird's nest, caught a trout, or done
any other boyish trick. The king said to him, 'And did you do it well ? '
Oh yes, capitally. He spoke the truth, no doubt, for he dared not have
trified with the king; but the affair created hardly any interest.
Travel in tropical west Africa is a lurid tale of barbaric negro
states, of slave-hunting and human sacrifice, of monstrous animals
and pestiferous swamps, of mysterious rivers and dangerous forests,
of trading and carousing in the midst of pestilence and death, of
explorers devoting health and life to their zeal for observation and
for science. Among those whose lives were sacrificed to their
passion for west African travel there are two whose literary power
raises their books above the rest. These are W. Winwood Reade
and Mary Kingsley. Reade, a nephew of the novelist, was himself
a man of literary power and promise who gave his fortune and life
to west Africa. His African Sketch-book, a charming record
of three journeys, appeared in 1873. Not long after its publication,
its writer died from the effects of his share in the Ashantee
campaign. Mary Kingsley, whose father and two uncles were all
notable voyagers and authors, travelled for scientific observation,
In 1900 she died at Simon's Town of enteric fever, caught in
tending Boer prisoners. Her Travels in West Africa, though
marred in parts by overlaboured humour, is very good at its best:
On first entering the great grim twilight regions of the forest, you hardly see
anything but the vast column-like grey tree stems in their countless thousands
around you, and the sparsely vegetated ground beneath. But day by day, as
you get trained to your surroundings, you see more and more, and a whole
world grows up gradually out of the gloom before your eyes. . . . Nor indeed
do I recommend African forest life to anyone. Unless you are interested in it
and fall under its charm, it is the most awful life in death imaginable. And
if you do fall under its spell, it takes the colour out of other kinds of living.
One kind of travel, namely Alpine climbing, has produced a
copious modern literature-peculiarly British in character—which
scarcely goes farther back than the middle of the nineteenth
century. Peaks, passes and glaciers, a series of episodes described
by different writers, appeared in 1859. The play-ground of
Europe by Sir Leslie Stephen is marked by a peculiar literary
## p. 255 (#285) ############################################
VII] Travel in Poetry and Romance 255
distinction. Whymper's books on the Alps and on the Andes
provide plenty of exciting matter. Alpine writing, including the
works of living writers and also the pages of The Alpine Journal,
is generally of good literary quality, being largely the work of
accomplished men whose recreation is Alpine climbing.
The growth of the British oversea dominions has produced
many books of travel. Conspicuous among them are Sir Charles
Dilke's two books Greater Britain (1868) and Problems of Greater
Britain (1890) which contain the observations of two journeys in
America and the Antipodes. They are notable both for their
lucid, easy mode of expression, and still more for their political
insight and clear perception of immediate difficulties and of future
possibilities—possibilities which have since, in great part, been
realised.
Only actual books of travel have here been mentioned. It
would pass the scope of this chapter to do more than hint at the
influence of these books and of personal travelling reminiscences
upon English poetry and prose fiction. Defoe's Robinson
Crusoe, Swift's Gulliver's Travels, Coleridge's Ancient Mariner,
Michael Scott's Tom Cringle's Log, Charles Kingsley's West-
ward Ho! , Charles Reade's The Cloister and the Hearth, R. L.
Stevenson's Treasure Island, are typical examples, and the
list might be endlessly extended. Every poet of the nineteenth
century, from Wordsworth to Tennyson and Browning, has left
upon his pages some impression of his travels. From Fielding
to Stevenson one may dip into the novelists almost at random
to find sketches of travel. The first chapter of Guy Mannering is
a vivid picture of a Scottish journey. Tom Jones and Humphrey
Clinker take us along the country roads of England. Vanity Fair
gives a picture of continental travel before the days of railways:
Pickwick is fresh with the more homely humours of the English
roadside and coaching inn. Upon another plane, Charles Lever's
wanderings inspire his pen. Later literature abounds with smaller
books of the same family-fictitious or half-fictitious stories of
trips on foot or bicycle, in canoe or caravan, at home and abroad.
One other reflection occurs. Although the literature of travel
is not the highest kind, and, indeed, cannot be called a distinct
branch, of literature, yet a history of English literature rightly
assigns a space apart to such books, because this kind of writing,
perhaps more than any other, both expresses and influences
national predilections and national character. In view of the
a
## p. 256 (#286) ############################################
256 Literature of Travel, 1700—1900 ([
CH. VII
magnificent achievements and splendid records of other nations
who have preceded or accompanied the British in the fields of
travel and discovery, it would be most inappropriate to attempt
any kind of national comparison. But books of travel and books
inspired by travel have, probably, been more read in Great Britain
than any other books except novels. The educational value of
pleasant travel-books is great. They have provided the substance
of a thousand books for boys; and thus, both directly and in-
directly, have guided and fired the inclinations of many generations
of boys. And every reader, whether boy or man, finds in his
favourite books of travel some image of himself and some hint
towards moulding himself.
## p. 257 (#287) ############################################
CHAPTER VIII
THE LITERATURE OF SCIENCE
A.
PHYSICS AND MATHEMATICS
course.
THE brilliant achievements of British mathematicians, astro-
nomers and physicists under the influence of Isaac Newton
were followed by a long period of comparative inactivity. This
was largely due to the fact that, during a considerable part of the
eighteenth century, members of the British school were, more or less,
out of touch with their continental contemporaries. A free exchange
of views is essential to vigour and, the more varied the outlook
and training of those concerned, the more fruitful is the inter-
The effect of this isolation, moreover, was intensified by
the manner in which English writers strove in their demonstrations
to follow Newtonian forms. If Newton, in his Principia, confined
himself to geometrical proofs, it was because their validity was
unimpeachable; and, since his results were novel, he did not wish
the discussion as to their truth to turn on the methods used to
demonstrate them. But his followers, long after the principles of
the calculus had been accepted, continued to employ geometrical
proofs, whenever it was possible, even when these did not offer
the simplest and most direct way of arriving at the result.
In short, we may say that, in the course of English mathe-
matical science, the last seventy years of the eighteenth century
form a sort of isolated backwater; for this reason, it is unnecessary
here to describe in detail the work of the writers of this period.
We must not, however, fall into the error of thinking that, among
them, there were no men of ability. The investigations of Colin
Maclaurin, of Edinburgh, on attractions, are excellent, and his
treatise on fluxions is, perhaps, the best exposition of that method
of analysis. We may also refer to the work of Thomas Simpson,
of London, on the figure of the earth, tides and various astro-
nomical problems; of John Michell, of Cambridge, who determined
the law of force between magnetic poles, invented the torsion
17
a
E. L. XIV.
CH. VIII.
## p. 258 (#288) ############################################
258 The Literature of Science [CH.
balance and devised the plan of determining the density of the
earth carried out by Cavendish in 1798; of Henry Cavendish",
who discovered the law of attraction in static electricity, introduced
the ideas of electrostatic capacity and specific inductive capacity
and determined the density of the earth by his wellknown
experiments; and of Joseph Priestley! , who also discovered, in-
dependently of others, the law of attraction in electrostatics and
the existence of oxygen ; while, in observational astronomy, we
need only refer to the great achievements of James Bradley and
(Sir) William Herschel. In applications of science, this period
and the early years of the nineteenth century were notable for the
development of the steam-engine. Somewhat earlier, Thomas
Savery and Thomas Newcomen had done much to bring it into
practical use; but modern forms may be said to date from the
improvements introduced by James Watt, Richard Trevithick and
Henry Bell.
With the nineteenth century, a new era in the history of
mathematics and theoretical physics in Great Britain opened.
We shall deal here only with its main features, and, so far as
possible, shall avoid technical details. Unfortunately, limits of
space forbid the introduction of those biographical touches which
would have added to the human interest of the story we have
to tell.
The first thirty or thirty-five years of this period were largely
occupied with work preparatory to the outburst of activity that
characterised the Victorian renascence. Early in the nineteenth
century, the use of analytical methods was introduced in the
Cambridge mathematical curriculum. The advocacy of this change,
originated by Robert Woodhouse, was warmly taken up by George
Peacock, Charles Babbage, (Sir) John Herschel, William Whewell
and (Sir) George Airy. These men worked under the influence of
the great French school, of which Lagrange and Laplace are the
most prominent members, and were hardly affected by their con-
temporaries, such as Gauss, Abel and Jacobi, who were then creating
new branches of pure mathematics. In England, at the beginning
of the century, Cambridge was recognised as the principal mathe-
matical school: all the reformers were residents there, and they
directed their efforts mainly to the introduction of a free use
of analysis in the university course of study. They were
successful ; and, by 1830, the fluxional and geometrical methods
of the eighteenth century had fallen into disuse. The leadership
1 See section B of the present chapter.
## p. 259 (#289) ############################################
Vill] Royal Institution. British Association 259
of Cambridge in this change was undisputed, and the employment
of analytical methods became usual throughout Great Britain.
In these years, a good deal of interesting work in physics and
chemistry was done in London, where the Royal Institution in its
laboratories offered far better opportunities for research than any
similar body in Britain. In connection with this society, we may
mention the work of Thomas Young, whose investigations on wave
motion prepared the way for the acceptance of the undulatory
theory of light, and we may associate with him the names of
(Count) Rumford and (Sir) David Brewster; optics and heat being
the subjects to which their especial attention was directed. At
the same time, John Dalton', in Manchester, was studying the
expansion of gases under varying changes of pressure and
temperature, and the tension of vapours.
At this time, interest in natural philosophy was widely dissemi-
nated, and, in science, as in politics and literature, new ideas were
readily welcomed. Institutes and scientific societies were founded
everywhere, and popular lectures by experts spread broadcast
general, though somewhat vague, information on natural philosophy
and astronomy. The year 1831 is memorable for the foundation
of the British Association for the Advancement of Science. The
intention of its promoters was that the Association should meet
every year for a few days at a provincial town under a distin-
guished president, with the object, partly, of encouraging personal
intercourse between leading men of science and, partly, of pro-
moting interest in scientific work in the various localities where
meetings were held. The meetings led to the regular appointment
of expert committees instructed to report on the progress in various
subjects; these reports have been, and are, of permanent value.
By way of addition to this preliminary statement, we may
also, in passing, mention the History of the Inductive Sciences,
published by Whewell in 1837. It put together in a readable
form the leading facts connected with the history and growth of
science, and, though open to criticism on questions of details-
as was inevitable in the case of an encyclopaedic work of the
kind-it served a useful purpose. Hardly less important was The
Penny Cyclopaedia, issued in twenty-seven volumes in 1833—43
with three supplements.
The most notable physicist at the beginning of the Victorian
period was Michael Faraday), who, in 1831, had begun those
investigations on electricity which have altered our conceptions
1 See section B of the present chapter.
17-2
## p. 260 (#290) ############################################
260
[CH.
The Literature of Science
of the subject, and, by their applications, have revolutionised
industrial science. Faraday had been brought up in humble
circumstances, and his career is interesting as an illustration of
the fact that, in England, no door is closed to genius. In 1812,
after attending some lectures delivered by Sir Humphry Davy,
'he sent notes of them to Davy, asking his assistance to enable him
to study science. The result was that Davy employed him as an
assistant in the chemical laboratory in the Royal Institution.
Here, Faraday's experimental skill soon led to appreciation of his
powers, and he wrote various papers on scientific questions.
Faraday's earliest electrical work related to induced currents,
and depended on his discovery of the fact that, if a wire in the shape
of a closed curve is moved to or from another wire through which
an electric current is flowing, a current is set up in the former
wire which ceases so soon as the motion ceases. The induced
current is caused by and depends on the motion of the one
wire relative to the other. Magnetic effects can be similarly
produced. Faraday went on to explain various phenomena by
the action of the induced currents which he had discovered.
As he pondered on possible explanations of these results, it
occurred to him that all space might be filled by lines of
magnetic force, every line being a closed curve passing through
the magnet to which it belongs ; and he pointed out that the
existence of these lines was suggested by the familiar experi-
ment of the arrangement of iron filings in such lines about a
magnet from whose poles they radiate. According to this view, ,
these induced currents were caused by the closed wire (or any
conductor) being moved across lines of force in its plane of motion,
and, if so, the electromotive force of an induced current would be
proportional to the number of unit lines of magnetic force cut in a
second by the moving wire. Now, the earth itself may be regarded
as a gigantic magnet, and, hence, if a copper wire spin across the
earth's lines of force, we should expect currents to be produced.
This was found to be the case. By these experiments, Faraday
tapped vast and hitherto unknown sources of electricity. The
use of dynamos as a source of mechanical power resulted from
these discoveries.
These investigations were followed by experiments to show
the identity in kind of electrical currents, however produced.
His investigations on electrolysis attracted general attention to
the subject, and led him to the remarkable conclusion that there
is a certain absolute quantity of electricity associated with each
## p. 261 (#291) ############################################
VIII]
Faraday
261
atom of matter. A few years later, in 1845, he discovered another
remarkable series of phenomena dependent on the fact that the
plane of polarisation of light can be rotated by the action of
magnets and electric currents; and, somewhat later, he discovered
and investigated diamagnetic properties in bodies.
The provision of well equipped laboratories is a modern
luxury, and Faraday was exceptionally fortunate in having access
to one. It is difficult to overrate his abilities as an experimental
philosopher; and, though he knew but little mathematics, his
conception of lines of force was essentially mathematical, and
was developed later by Clerk Maxwell and other writers. At the
time, however, it repelled mathematicians accustomed to the
formulae and symbols with which Laplace and Poisson had made
them familiar. It is interesting to see that Faraday, like Newton,
refused to contemplate the possibility of action at a distance, but
sought, rather, to explain the phenomena of attraction by changes
in a continuous medium. He was followed at the Royal Institution
by John Tyndall, whose lectures did much to excite and maintain
general interest in physical questions.
While Faraday was opening new ways of regarding physical
phenomena, the classical methods of Poisson were being applied
with success by James MacCullagh, of Dublin, to problems of
physical optics. In these investigations, MacCullagh, like his
continental contemporaries, elaborated the conception of the
ether as an elastic solid, and, thence, he deduced the laws of re-
flection and refraction ; but, though his work was ingenious, many
of his conclusions were vitiated by his erroneous assumption that
the vibrations of plane polarised light are parallel to the plane of
polarisation. Another physicist of this time whose work has
been of importance was James Prescott Joule, a pupil of Dalton,
who showed that heat and energy were interchangeable in definite
proportions. Mention should also be made of (Sir) Charles
Wheatstone, who, about 1810, brought electric telegraphy into
general use. Wheatstone was a man of wide interests : he early
suggested the use of spectrum analysis for chemical researches
invented stereoscopic instruments and, later, did much useful work
in the construction of dynamos.
This period was rich in inventions whereby science was applied
practically, as, for example, the general employment of steam-
engines for locomotion, the electric telegraph and the introduction
of lighting and heating by gas.
We turn from these practical applications to consider more
## p. 262 (#292) ############################################
262 The Literature of Science [CH.
abstract researches. Faraday was recognised as an exceptional
genius, and time has strengthened the recognition of his claim to
distinction ; but, in general, theoretical physics had, by now,
become so closely connected with mathematics that it seemed
hardly possible for anyone without mathematical knowledge to
make further advances in its problems. This association lasted
well into the twentieth century, and the continuation and extension
of Faraday's work fell into the hands of mathematicians.
Before proceeding to describe the remarkable work of the
school of mathematical physicists who followed Faraday, it will be
convenient to mention the leading writers of this time on pure
mathematics. We may begin by noting the fact that the range
of pure mathematics had, ere this, grown to an extent which
rendered it difficult for any man to master more than a compara-
tively small section of it, and, a fortiori, physicists took up only such
special branches of mathematics as were required for their own
purposes. We should also notice that one of the striking features
of this period has been the largely increased number of students
of mathematical and physical science: hence, to mention only
the leading writers does indirect injustice to others whose work,
though not epoch-making, has been of real importance. With
this caution, we proceed to name a few of those whose researches
have permanently affected the development of mathematics.
In the period on which we are now entering, we find half-a-
dozen mathematicians—De Morgan, Hamilton, Sylvester, Adams,
Cayley and Smith—whose researches will always make it memor-
able. Hamilton and Smith were fastidious writers, and, apart
from the value of their work, it is a pleasure to observe the
artistic manner in which they presented it; but their pupils
were few, and it was only to a select number of scholars that their
writings appealed. The others were more fortunate in being
connected with the great mathematical school of Cambridge.
Their methods are sharply contrasted. De Morgan wrote vi-
vaciously, and largely for non-specialists. Cayley's writings were
precise and methodical, and he always sought to be exhaustive.
Sylvester's papers, like his lectures, were badly constructed,
impetuous and often unfinished ; yet, experience proved them to
be amazingly stimulating. Adams's work was elegant and highly
polished. Modern pure mathematics deals so largely with abstract
and special subjects that it is almost impossible to describe the con-
clusions in a way intelligible to laymen. It will suffice to indicate
the subjects of their principal researches.
## p. 263 (#293) ############################################
vi]
Pure Mathematics
263
Of these mathematicians, Augustus De Morgan was the oldest.
He was educated at Cambridge, but, at that time, office in the
university was conditional on certain declarations of religious
belief. In consequence of this, he moved to London, and there,
through his writings and lectures, exercised wide influence. He
was well read in the philosophy and history of mathematics ; but
it is on the general influence he exerted rather than on dis-
coveries of his own that his reputation rests. With his name
we may associate that of George Boole, of Cork, the creator of
certain branches of symbolic logic, whose mathematical works are
enriched by discussions on the fundamental principles of the
subject. His writings are valuable in themselves, and their pre-
sentment of conclusions is lucid and interesting.
(Sir) William Rowan Hamilton was among the first of a small
but brilliant school of mathematicians connected with Trinity
college, Dublin, where he spent his life. We regard his papers
on optics and dynamics as specially characteristic of his clearness
of exposition: theoretical dynamics being properly treated as a
branch of pure mathematics. He is, however, best known by his
introduction, in 1852, of quaternions as a method of analysis.
Hamilton, followed, later, by authorities so good as P. G. Tait of
Cambridge and Edinburgh, A. Macfarlane of Edinburgh and
Pennsylvania and C. J. Joly of Dublin, asserted that this would
be found to be a potent instrument of research; but, as a matter
of fact, though it lends itself to concise and elegant demonstra-
tions, it is but little used by mathematicians today. In connection
with Dublin, at this time, we must also mention the name of
George Salmon, provost of Trinity college, whose works on ana-
lytical geometry and higher algebra are classical examples of how
advanced text-books should be written, and that of (Sir) Robert
Stawell Ball, first, of Dublin and, later, of Cambridge, who
produced a classical treatise on the theory of screws.
James Joseph Sylvester, like De Morgan, found an academic
life at Cambridge denied him in consequence of his theological
tenets ; but the subsequent abolition of religious tests at the older
universities enabled him, towards the end of his life, to accept
a chair at Oxford. He was a prolific writer; perhaps his favourite
studies were the theory of numbers and higher algebra ; in the
latter subject, he dealt especially with canonical forms, contra-
variants and reciprocants. The lectures that he gave at Baltimore
from 1877 to 1883, did much to stimulate interest in pure
mathematics in America.
7
## p. 264 (#294) ############################################
264
The Literature of Science
[CH.
John Couch Adams was another graduate of Cambridge, and
spent all his life in that university. There are three important
questions in theoretical astronomy, treated as a branch of pure
mathematics, which are especially connected with his name. The
first of these is his discovery, in 1846, of the planet Neptune,
through the disturbance caused by it in the orbit of Uranus ; this
was made independently of, and a few months earlier than, the
similar investigation by Leverrier. This finding of an unsuspected
and unseen planet afforded a striking demonstration of the uni-
versality of gravitation, and excited widespread admiration. The
second of these famous investigations is to be found in Adams's
discussion, published in 1855, of the secular acceleration of
the moon's mean motion—a difficult problem, involving heavy
analytical work and elaborate historical enquiries. The third is
his determination, in 1867, of the orbit of the Leonid shooting
stars.
Arthur Cayley, likewise, spent the bulk of his life at Cambridge,
first as a student and then as a professor. He discussed many
subjects in pure mathematics, his most notable researches dealing
with the general theory of curves and surfaces in analyticalgeometry,
with the theory of invariants in higher algebra, and, in ten classical
memoirs, with binary and ternary forms. He also wrote at length
on elliptic functions, but treated it from Jacobi's point of view;
and, in consequence of Weierstrass's work, much of this is out
of date.
Henry John Stephen Smith, who was educated at the sister
university of Oxford, will be long remembered for his work on the
theory of numbers, especially on linear determinate equations, and
the orders and genera of ternary quadratic forms. He was a
graceful lecturer and writer ; but, while the value of his researches
was recognised, he founded no school. His paper on the repre-
sentation of numbers by sums of four, six, eight, five and seven
squares was the occasion of a curious incident, which illustrates,
incidentally, the widespread ignorance of his work. Fourteen
years after it had been published in the Proceedings of the
Royal Society, the problem, for the single case of five squares, was
proposed by the French Academy as a subject for its grand prize,
open to the world. The problem had, in fact, years before, been
completely solved by Smith, who, to secure the reward had only
to write out his demonstration for the special case proposed.
We have already briefly described Adams's investigations in
mathematical astronomy, and, perhaps, we may here add a word
## p. 265 (#295) ############################################
VII]
Theoretical Physics
265
or two on the researches of (Sir) George Howard Darwin, also of
Cambridge, who investigated the form taken by a rotating viscous
mass of matter, and showed that, in the early history of the solar
system, the moon arose from a portion of the earth thrown off
(when the latter was in a plastic condition) through its increasing
velocity of rotation. Later, he demonstrated that the moons of
the other planets could not have originated in the same way. He
wrote at length on the theory of tides. He also worked at the
problem of three bodies, investigating, by lengthy arithmetical
methods, possible stable forms of periodic orbits of one body,
moving under the attraction of two other bodies.
With observational and practical astronomy we are not here
concerned; but we may add that the results of the astronomical
discoveries of the Victorian period were made familiar to the
English speaking world by the popular treatises and lectures of
Sir Robert S. Ball whom we have already mentioned, and by
various works by Miss A. M. Clerke.
Mention may here be made, also, of two great teachers of the
Victorian age, to wit, William Hopkins, and Edward John Routh,
under whom many generations of Cambridge mathematicians were
educated, and to whom the predominance in Britain, throughout
the period here treated, of the mathematical school of that university
is largely due. Of more recent English writers on pure mathematics,
some have devoted themselves to higher analysis, especially
differential equations, differential geometry and the theory of
functions ; others have followed continental initiative in discuss-
}
ing the fundamental principles and philosophy of mathematics.
We return to the subject of theoretical physics.
It was the
good fortune of the Cambridge school to produce, in the Victorian
period, some of the greatest physicists of the century. The
university course for a degree, at that time, involved a study of
the elements of nearly all the branches of mathematics then read;
and, thus, its graduates were exceptionally well equipped for
discussing physical problems from the mathematical side. Among
these physicists, we here mention briefly the work of George Green,
(Sir) George Stokes, (Sir) William Thomson afterwards lord Kelvin,
and Clerk Maxwell. To their credit, be it said, they all treated
symbols and formulae as servants and not as ends in themselves.
George Green was a self-educated man, who came to Cambridge
in middle life and took his degree in 1837, unfortunately for science
dying four years later. In 1828, he introduced the idea of the
potential, representing the work which must be done to move a
## p. 266 (#296) ############################################
266 The Literature of Science [CH.
unit of mass from infinity to its position. In this memoir is
established the celebrated formula, connecting surface and volume
integrals, which forms a fundamental proposition in the theory of
attractions. Green wrote on various physical questions, notably
on the motion of waves in a canal, and the deduction of the
geometrical laws of sound and light from the undulatory theory.
In these writings, he showed remarkable physical insight in the
applications of his analysis. His memoirs on the propagation of
light in a crystalline medium, published in 1839, rest on the
assumption that the ether in a crystal resembles an elastic solid
unequally pressed in different directions by unmoved ponderable
matter—a conception which, later, was to lead to remarkable
developments. Few writings have been more fruitful than those
of Green. They led MacCullagh and Cauchy to revise their theories
of optics, and they profoundly impressed Stokes and Kelvin, whose
work we now proceed to describe.
(Sir) George Gabriel Stokes spent his life at Cambridge, where
he held the Lucasian chair for over half a century. Through his
long tenure of the secretaryship of the Royal society, he acted as
the friend and guide of innumerable young authors, for, by virtue
of his office, he saw the manuscripts of all papers on mathematics
and physics, and freely placed at the disposal of the writers his
unrivalled knowledge of physics and mathematics : thus, a con-
siderable proportion of his work appears under the names of other
writers. He began his scientific career under the influence of
Green's writings. It is difficult to describe his researches in
general terms. The most important of them are concerned with
optics, hydrodynamics and geodesy. In optics, he was mainly
responsible for the explanation of fluorescence, and only just
missed being the first to propound the true explanation of
Fraunhofer's lines; he subjected diffraction to mathematical
analysis ; in hydrodynamics, we owe to him the modern theory
of viscous fluids, and he wrote on the properties and constitution
of the ether. His work in pure mathematics, especially on the
convergence of series, was also of importance. Stokes was an
excellent man of affairs-he sat for a time in the house of
commons—but his gift of silence prevented his exercising among
strangers the full influence which his abilities deserved. He was
the intimate friend of Kelvin and Maxwell, and to his deliberate
judgment on scientific matters Kelvin always yielded.
(Sir) William Thomson, later raised to the peerage under the
title baron Kelvin, was another graduate of Cambridge of this
## p. 267 (#297) ############################################
VIII]
Kelvin
267
period. (To avoid the confusion of the use of two names we will
here refer to him as Kelvin. ) In 1846, the year following on
his first degree, he accepted a professorial chair at Glasgow, but
he always kept in touch with his mathematical friends in Cambridge.
Probably, he exercised a wider influence in the world at large
than any of his scientific contemporaries; but his interests were so
catholic that it is not easy to give any connected account of them.
He possessed an almost intuitive power of realising fundamental
principles. Throughout his life, ideas seem to have come to him
so rapidly as to give him insufficient time for their effective
development; hence, the student will search in vain among his
papers for complete and systematic expositions of his discoveries.
He began his career under the influence of Fourier, Green and
Faraday. Electricity was his favourite subject of research. The
writer of this sketch has heard him illustrate the progress in this
subject by the fact that, in his early life, he was accustomed to
explain his conclusions in it by analogies drawn from the theory
of heat; but, before he died, problems in heat were commonly
illustrated by analogous questions in electricity. Kelvin wrote
at length on the subject of electromagnetic fields, put forward
numerous suggestions about the constitution of ether and matter,
and laid the foundation for a scientific system of measurement of
electrical quantities. · Hydrodynamics, elasticity and thermo-
dynamics were other subjects on which he wrote, and his papers
on energy and entropy were of far-reaching importance. We
cannot leave Kelvin's work without mentioning the appearance,
n 1867, of the treatise on natural philosophy by himself and his
friend Peter Guthrie Tait, of Cambridge and Edinburgh. In spite
of Tait's collaboration, this book presents an unfinished aspect;
but it is suggestive, and it widely affected modes of physical
thought throughout Europe.
The theory of signalling by cable originated in a correspondence
between Kelvin and Stokes in 1854, and was elaborated by Kelvin
and Kirchhoff, while to the former are largely due the practical
applications of it. The earliest successful submarine cable laid
between England and the continent dates only from 1851. Owing
to the large capacity of the cable and the soakage into the
insulating material, powerful currents had to be used before
sensible effects could be obtained, and these difficulties increased
with the length of the cable. Accordingly, when, in 1857, a cable
was laid to America, the operators deemed it necessary to use
electricity of a high tension, with the result that the insulation
## p. 268 (#298) ############################################
268 The Literature of Science [Ch.
a
was ruptured. Subsequently, Kelvin was given a free hand in
arranging a system for use with a later cable. He evaded the old
difficulties by developing extreme sensitiveness in the receiving
instruments and applied the methods of Gauss and Weber for
indicating the minute motions of the oscillating needle by the
reflection of a ray of light from it, in effect employing a long
non-material pointer. In 1870, he substituted for this method
a syphon-recorder which printed the message ; and this instrument
is still sometimes used. He held that the transmission of signals
along the wire of a submarine cable was due to an actual dis-
turbance in the wire : whereas, according to the modern theory,
propounded by Maxwell, the function of the wire is merely to
guide the disturbance resident in the surrounding dielectric.
Kelvin was a keen yachtsman, and was thus led to take up the
problem of compasses ; he also bore an active part in the develop-
ment of electrical engineering. He was the owner of several
patents connected with these practical applications of science.
We have next to mention one whose work has had so important
an influence on the subsequent growth of the subject as to make
it the beginning of a new epoch. This was James Clerk Maxwell
-the most modest of men-another member of the Cambridge
school, who, for the last eight years of his life, occupied
in the university the then newly created chair of experimental
physics.
Since the time of Descartes, natural philosophers had never
ceased to speculate on the processes by which gravity, light and
electricity are transmitted through space. So far as electricity
is concerned, the idea of lines of force in a continuous medium is
due to Faraday. Kelvin, as a young man, had suggested that
electric force might be transmitted through a medium, somewhat
as elastic displacements are transmitted through an elastic solid.
This idea was taken up by Maxwell, who, in 1856, elaborated the
analogies offered by the flow of a liquid, and, five years later,
devised a mechanical model of electromagnetic action. He now
brought forward a series of arguments to show that an electric
current was a phenomenon of translation, magnetism one of
rotation and the electrostatic state one of strain of the ether.
These conclusions led him to assert that light consists of trans-
verse waves of the same medium as that required for the
explanation of electric and magnetic phenomena. On this theory,
all currents are closed; magnetic energy is the kinetic energy
of the ether, and electric energy the energy of 'strain of the ether.
## p. 269 (#299) ############################################
VIII)
Maxwell's Electromagnetic Theory 269
These views were presented, as a whole, in 1864. Further ex-
tensions and developments of the theory followed, and the whole
was set out in his treatise published in 1873. This celebrated
work is far from easy to read, and the exposition is not
systematic, but it may be said that the fundamental ideas are now
universally accepted, and most of the work of his successors has
been built on the foundation here laid. The theory was based on
Faraday's ideas; but it required a trained mathematician to give
the final form to his conceptions and to deduce their consequences.
Hence, the theory is properly associated with Maxwell's name.
Maxwell, also, took a considerable part in framing a standard
system of electrical measurements. He contributed largely to the
kinetic theory of gases, and, incidentally, to theories on the con-
stitution of matter.
A large part of the history of mathematical physics during the
last quarter of the nineteenth century consists of the completion
and extension of Maxwell's electromagnetic theory. No incon-
siderable part of this is due to his successors at Cambridge, and to
describe recent researches in physics without mentioning the names
of lord Rayleigh, Sir Joseph John Thomson and Sir Joseph Larmor
is almost impossible; here, however, we must content ourselves
with a very brief account of the general line of investigation
followed in the last part of the period covered by the present
section.
It has already been pointed out that Maxwell's exposition of
his electromagnetic theory of light was neither systematic nor
complete. A curious omission in it was the absence of any
explanation of reflection and refraction ; this was supplied by
Helmholtz. The problem of the effects produced by the translation
of electric charges, raised by the same investigator, was solved by
the researches of the present Cavendish professor at Cambridge,
George Francis FitzGerald of Dublin, and others : in the mathe-
matical development of the theory, which now proceeded apace,
they, again, took a prominent part. In 1883, FitzGerald explained
a system of magnetic oscillators by which radiant energy could
be obtained from electrical sources, thus confirming Maxwell's
theoretical conclusion that light was an electromagnetic phe-
Some of Maxwell's assumptions on which he had
based his theory still remained unconfirmed; but, a year or two
later, the theory was placed on a firmer experimental basis by
Hertz. The results, incidentally, led to the introduction of wire-
less telegraphy.
nomenon.
## p. 270 (#300) ############################################
270 The Literature of Science [CH.
The question of the conduction of electric discharges through
liquids and gases had been raised by Faraday. It was now taken
up seriously, and various types of rays, cathode rays, Röntgen
rays, etc. , were discovered. These researches led to new views on
the constitution of matter. The investigations began with a
theory of electrons, and, finally, led to the view that every so-
called atom is formed by a combination of two elements in varying
proportions, and that, possibly, these two elements are to be
identified with forms of electricity-one of the most far-reaching
hypotheses propounded in recent times.
The efforts to extend the theory of the electromagnetic field to
cases where heavy masses are in motion introduces the difficult
question as to whether the ether round and in bodies is affected
by their motion, and to this theory of relativity much attention
is now being paid.
One of the striking features of the Victorian period has been
the equipment of large laboratories where experiments can be
carried out by students with an accuracy wholly impossible in
former days. Two of the earliest of these were built at Oxford
and Cambridge, the former known as the Clarendon laboratory
in 1872, the latter, known as the Cavendish laboratory, being
the gift of the seventh duke of Devonshire. In the latter,
Clerk Maxwell taught and has been succeeded by professors
not less distinguished. The existence of such laboratories in
seats of learning has profoundly affected the teaching of the
subject by training large numbers of competent observers, besides
calling forth in ever widening circles an intelligent interest in
physical studies.
It is not, we think, too much to say that the work in physics of
the Victorian period has completely revolutionised the subject,
and, both on its theoretical and practical sides, far exceeds in value
that previously done in any period of similar extent. The theory
of gravitation was the great achievement of the Newtonian school.
In the following century, physical optics and, later, the nature of
ether attracted most attention from philosophers, while practical
men developed the steam-engine and studied the theory of heat.
The Victorian age has seen electricity raised to the rank of an all-
embracing science, and applied to innumerable industrial uses,
power-engines, lighting, heating, telegraphy, telephones. Other
important scientific and industrial applications relate to photo-
graphy and spectrum analysis ; the development of the turbine-
engine; the invention of the internal combustion-engine, with its
## p. 271 (#301) ############################################
VIII]
Chemistry
271
numerous uses in transport on land and water; the introduction
of submarine boats, and heavier-than-air flying-machines ; and
the use of wireless telegraphy. In this chapter, however, bare
reference to these practical applications must suffice.
B. CHEMISTRY
Chemistry has always busied itself with the changes of
material things. By working in metals and precious stones, by
making colours, by producing things used by artists to give
delight to themselves and others, by fashioning natural materials
into things useful to men, by concocting potions which had strange
effects on the bodies and minds of those who swallowed them, by
doing these things and things like these, chemists slowly amassed
much knowledge, knowledge, however, which was fragmentary and
disconnected. The strange changes which chemists discovered
impelled the more ardent and adventurous among them to dream
of the possibility of finding a universal medicine which should put
an end to disease and suffering and enable the adept to bring all
imperfect things to a state of perfection. The history of alchemy
is the history of a particular branch of the universal quest, the
quest of the unchanging.
In the later years of the eighteenth century, between 1770 and
1790, chemistry passed, at a bound, from being an empirical art to
becoming a science. The man who made the great transformation
was Antoine Laurent Lavoisier. With the work of the master we
are not concerned here.
From the time of Lavoisier to our own day, chemistry has
progressed, in the main, along four lines. For some years, chemists
concentrated their attention on one definite class of material
changes, the changes which happen when substances are burned
in the air. The knowledge which was gained of the changes of
composition and of properties during combustion incited and
guided chemists to a searching examination of the distinctive
properties of many different substances, and this examination
brought about the clarifying of the conception of definite kinds
of matter, and the application of this conception to the opening of
many paths of chemical enquiry. While these advances were being
made, a quiet member of the Society of Friends presented chemistry
with a marvellously delicate and penetrative instrument for further-
ing accurate knowledge of material changes. John Dalton made
## p. 272 (#302) ############################################
272
The Literature of Science [CH.
what seemed a small addition to the Greek atomic theory, an
addition which changed an interesting speculation into a scientific
theory. As the century went on, chemists began to elucidate
the connections between chemical events and physical phenomena.
The science of physical chemistry began.
Among those who investigated the phenomena of combustion
in the eighteenth, and early nineteenth, century, Priestley and
Cavendish are pre-eminent. Black was the first chemist to
make an accurate, quantitative examination of a particular,
limited, chemical change, and, by so doing, to give clearness to
the expression 'a homogeneous substance. ' The atomic theory
was Dalton's gift to science. From the many chemists who
amplified the work of Dalton, and used the conceptions of atom
and molecule to connect and explain new classes of chemical facts,
Williamson and Frankland may be selected as the representatives.
As workers in the borderland between chemistry and physics,
Graham and Faraday are specially to be remembered. The in-
vestigations of Davy touched and illuminated every side of
chemical progress.
Besides these men, who greatly enriched and advanced the
science of chemistry in the period under review, there were
many workers whose contributions cannot be considered here,
References are given in the bibliography to the writings of some
of them.
Joseph Priestley was a man of many gifts and a very versatile
mind. When a youth at an academy, he tells us that he 'saw
reason to embrace what is generally called the heterodox side of
almost every question. When about twenty-eight years of age,
he taught, in a school at Warrington, languages (he had a great
natural gift of tongues), oratory and criticism, elocution, logic,
natural phenomena, civil law and anatomy.
In the seventies of the eighteenth century, Priestley turned his
attention to different kinds of airs. He obtained and partially
examined many gases, but rarely troubled about separating them
completely from impurities. In August 1774, Priestley obtained a
large lens with which he concentrated the sun's rays on whatever
substance happened to come to his hand, with the object of finding
what air could be extracted from it. When he thus heated mer-
curius calcinatus per se (now called oxide of mercury), he obtained
an air in which a candle burned with a remarkably vigorous
flame. ' This result, he says, 'surprised me more than I can well
express. ' The new air was subjected to many tests; it always
6
## p. 273 (#303) ############################################
VIII] Priestley's Work on Gases
273
behaved in a very unexpected manner. He placed a mouse in his
new air; the mouse remained lively, and the air did not become
noxious. ' The results of other experiments caused Priestley
to lie awake through the night ‘in utter astonishment. ' At last,
he concluded that the new air was 'between four and five times as
good as common air. He regarded the new air as a very superior
kind of common air.
Priestley thought alchemically, not as a chemist. To the
alchemist, the properties of things were external wrappings which
might be removed from one thing and put round another, without
affecting the essential substance of either thing, which substance
it was the business of properties to hide from the uninitiated.
Priestley thought of different airs as identical, or nearly identical,
in substance, and only apparently different because of superficial
differences in the mantles, the properties, by which the essential
substance was concealed. When he obtained the air from burnt
mercury, he thought he had removed from common air something
which made it ‘noxious, vitiated, depraved, corrupt. ' He had not
learnt, what Black’s experiments, made twenty years before 1774,
might have taught him, that each particular, material thing is
known only by its properties. Priestley's forced explanation of the
facts which he himself discovered helped to convince investigators
that the notion of identity of substance hidden under differences
of properties is a great hindrance to the acquirement of accurate
knowledge of natural events.
Priestley could not get over his astonishment at the behaviour
of the new air. In science, one does well to be astonished; but,
to astonishment one must add investigation, to investigation,
reasoning, and, to reasoning, more investigation. Stopping at
astonishment, Priestley made his facts square with the theory that
dominated him, the theory of phlogiston. The phlogisteans taught
that something, which they had named phlogiston, the principle of
fire, rushes out of a burning substance as it burns. Phlogiston
was never captured. Priestley held that the elusive phlogiston is
a great corrupter of your airs or gases. He supposed that he had
deprived common air of this depraving principle; he named his
new gas dephlogisticated air. He invented many very ingenious
hypotheses to account for facts observed by himself. Had he
made a few accurate quantitative experiments, he might have
broken the toils of his favourite theory.
The French chemist Lavoisier saw the importance of Priestley's
discovery of dephlogisticated air, and, by a series of rigidly
18
E. L. XIV.
CH. VIII.
## p. 274 (#304) ############################################
274 The Literature of Science [ch.
>
quantitative experiments with tin and mercury, proved that, when
a substance burns in air, it combines with a constituent of the air,
which air-constituent is the gas prepared by Priestley. Lavoisier
called this gas oxygen, because many of its compounds are
acids.
Priestley's insatiable curiosity, his mental alertness, his im.
patience of details, were required for the advancement of chemistry,
no less than the passionless determination and the scrupulous
accuracy of Cavendish.
Henry Cavendish, of Peterhouse, was bred in the theory of
phlogiston, as Priestley was, and remained faithful to that theory,
as Priestley did. He thought of many airs, or gases, as more or less
phlogisticated forms of a few particular substances. Cavendish
described the explosion of a mixture of common air and inflammable
air (obtained by the action of acids on zinc) as one of the ways of
phlogisticating air. This process is accompanied by a decrease in
the volume of the interacting gases. Cavendish tried to discover
the cause of this decrease. He exploded accurately measured
volumes of dephlogisticated air (oxygen) and inflammable air
(hydrogen), and found that water was the sole product of the
change when dephlogisticated air was mixed with twice its volume
of inflammable air. The explanation which Cavendish gave of
this fundamentally important fact was confused and vague, because
he insisted on making the facts uphold the phlogistic theory.
Without knowing exactly what he had done, Cavendish had
determined the quantitative volumetric composition of water.
When the phlogistic theory had been swept away, the very
great importance of the accurate work of Cavendish became
manifest.
Joseph Black graduated as doctor of medicine in the university
of Edinburgh in 1755, presenting a thesis entitled Magnesia
alba, Quicklime, and other alcaline substances. That thesis is
probably the earliest example of a genuinely scientific chemical
investigation. Black proved that mild magnesia (now called
magnesium carbonate) loses weight when it is calcined; he deter-
mined the loss of weight; he proved that the solid substance
which remains after calcination has properties of its own which
distinguish it from mild magnesia; he showed that, during calci-
nation, an air, or gas, is given off, different from any air, or gas,
then known ; he examined, accurately, the properties of this gas,
which he called fixed air; and he reproduced the original quantity
of mild magnesia by dissolving the calcined magnesia in acid, and
>
## p. 275 (#305) ############################################
VIII) Black and Cavendish
275
adding fixed alkali (now called potassium carbonate), a substance
which he proved to give off fixed air when it is calcined. By his
experiments, Black proved mild magnesia to be composed of fixed
air united with calcined magnesia, and showed that each of these
three substances is a particular and definite kind of matter, dis-
tinguished from all other kinds of matter by constant qualities.
He also proved that the change which happens when chalk is
burned is exactly similar to the calcining of mild magnesia ; fixed
air is driven out of the chalk, and burnt lime—a perfectly
definite homogeneous substance-remains.
The work of Black prepared the way for the penetrative, ex-
perimental analysis of the phenomena of combustion ; it taught
chemists to use accurately observed properties of bodies as the
only means of distinguishing one body from another; it showed
that, if chemical investigation is to produce results of permanent
value, it must be quantitative; incidentally, by isolating and
examining fixed air, it began a new branch of chemistry, the study
of the changes of composition and properties which happen when
homogeneous gases interact.
Black and Cavendish were painstaking, methodical, un-
emotional, eminently clear-headed. Priestley was flighty, fitting
from one thing to another in his laboratory, always curious, never
working out his discoveries, unable to think chemically outside of
the theory which dominated him. Black, Cavendish and Priestley
greatly advanced the science of chemistry.
So long as chemists formed vague generalisations founded on
introspective speculations, they made little progress. It was by
concentrating their attention on a few limited occurrences, and
accurately examining these by quantitative experiments, that
chemists gradually gained clear conceptions which could be
directly used in the investigation of more complicated chemical
changes. “True genius,' Coleridge said, 'begins by generalising
and condensing; it ends in realising and expanding. ' The vague
generalising of the alchemists was followed by the condensing
work of Black and Cavendish, and by the suggestive discoveries of
Priestley. The time was approaching for realising and expanding.
In 1808, a small book appeared, entitled A new system of
Chemical Philosophy, Part I, by John Dalton. The influence of
that book on the development of chemistry, and of physics also,
has been very great.
Dalton delivered a lecture in Manchester, in 1803, wherein he
said 'An enquiry into the relative weights of the ultimate particles
18-2
## p. 276 (#306) ############################################
276
The Literature of Science [CH.
of bodies is a subject, so far as I know, entirely new; I have lately
been prosecuting this enquiry with remarkable success. ' Many of
Dalton's predecessors, both chemists and physicists, had used, in a
vague and general manner, the Greek conception of the atomic
structure of matter. Dalton showed how the relative weights of
atoms can be determined. By doing that, he brought down the
atomic theory to the solid earth, and made it a bold, suggestive,
stimulating guide ready for the use of chemists and physicists.
Dalton was not a great experimenter; he generally used the
results of other chemists' experiments. He was a scientific thinker,
characterised by boldness and caution. Dalton assumed, as
Lucretius had done long before him, that matter has a grained
structure ; that all the ultimate particles of each particular
homogeneous substance are identical, and differ in properties,
one of which is their weight, from the particles of all other
definite substances; he also assumed that the mechanism of
chemical changes, that is, changes wherein homogeneous substances
are produced different from those present when the changes began,
is the coalescence of atoms of different kinds to form new sorts of
atoms.
In order to find the relative weights of atoms, Dalton argued
as follows: Analyses and syntheses of water show that eight
grains of oxygen unite with one grain of hydrogen to form water.
If this change is the union of atoms of oxygen with atoms of
hydrogen, to form atoms of water, and if all the atoms of each
one of these three homogeneous substances are identical in weight
and other properties, it follows that an atom of oxygen is eight
times heavier than an atom of hydrogen. If we take the atomic
weight of hydrogen as unity—because hydrogen is lighter than
any other known substance—then the atomic weight of oxygen is
eight, and the atomic weight of water is nine.
In arriving at the conclusion that the atomic weight of oxygen
is eight, if the atomic weight of hydrogen is one, Dalton made the
assumption that a single atom of oxygen unites with one atom of
hydrogen to form an atom of water. He made this assumption
because it was simpler than any other. Had he chosen to suppose
that two atoms of hydrogen unite with one atom of oxygen, he
must have assigned to oxygen the atomic weight sixteen, and to
water the atomic weight eighteen.
To make Dalton's method perfectly general, and quite conclusive
in its results, it was necessary to find means for fixing the relative
weights of the atoms formed by the union of other, simpler, atoms;
## p. 277 (#307) ############################################
VII] The Atomic Theory. Dalton and Others 277
it was also necessary to find means of determining the number of
atoms of each kind which unite to form a more complex atom.
own hands, and giving it full cock to a page, told him to go out and shoot
a man in the outer court; which was no sooner accomplished than the little
urchin returned to announce his success with a look of glee such as one would
see in the face of a boy who had robbed a bird's nest, caught a trout, or done
any other boyish trick. The king said to him, 'And did you do it well ? '
Oh yes, capitally. He spoke the truth, no doubt, for he dared not have
trified with the king; but the affair created hardly any interest.
Travel in tropical west Africa is a lurid tale of barbaric negro
states, of slave-hunting and human sacrifice, of monstrous animals
and pestiferous swamps, of mysterious rivers and dangerous forests,
of trading and carousing in the midst of pestilence and death, of
explorers devoting health and life to their zeal for observation and
for science. Among those whose lives were sacrificed to their
passion for west African travel there are two whose literary power
raises their books above the rest. These are W. Winwood Reade
and Mary Kingsley. Reade, a nephew of the novelist, was himself
a man of literary power and promise who gave his fortune and life
to west Africa. His African Sketch-book, a charming record
of three journeys, appeared in 1873. Not long after its publication,
its writer died from the effects of his share in the Ashantee
campaign. Mary Kingsley, whose father and two uncles were all
notable voyagers and authors, travelled for scientific observation,
In 1900 she died at Simon's Town of enteric fever, caught in
tending Boer prisoners. Her Travels in West Africa, though
marred in parts by overlaboured humour, is very good at its best:
On first entering the great grim twilight regions of the forest, you hardly see
anything but the vast column-like grey tree stems in their countless thousands
around you, and the sparsely vegetated ground beneath. But day by day, as
you get trained to your surroundings, you see more and more, and a whole
world grows up gradually out of the gloom before your eyes. . . . Nor indeed
do I recommend African forest life to anyone. Unless you are interested in it
and fall under its charm, it is the most awful life in death imaginable. And
if you do fall under its spell, it takes the colour out of other kinds of living.
One kind of travel, namely Alpine climbing, has produced a
copious modern literature-peculiarly British in character—which
scarcely goes farther back than the middle of the nineteenth
century. Peaks, passes and glaciers, a series of episodes described
by different writers, appeared in 1859. The play-ground of
Europe by Sir Leslie Stephen is marked by a peculiar literary
## p. 255 (#285) ############################################
VII] Travel in Poetry and Romance 255
distinction. Whymper's books on the Alps and on the Andes
provide plenty of exciting matter. Alpine writing, including the
works of living writers and also the pages of The Alpine Journal,
is generally of good literary quality, being largely the work of
accomplished men whose recreation is Alpine climbing.
The growth of the British oversea dominions has produced
many books of travel. Conspicuous among them are Sir Charles
Dilke's two books Greater Britain (1868) and Problems of Greater
Britain (1890) which contain the observations of two journeys in
America and the Antipodes. They are notable both for their
lucid, easy mode of expression, and still more for their political
insight and clear perception of immediate difficulties and of future
possibilities—possibilities which have since, in great part, been
realised.
Only actual books of travel have here been mentioned. It
would pass the scope of this chapter to do more than hint at the
influence of these books and of personal travelling reminiscences
upon English poetry and prose fiction. Defoe's Robinson
Crusoe, Swift's Gulliver's Travels, Coleridge's Ancient Mariner,
Michael Scott's Tom Cringle's Log, Charles Kingsley's West-
ward Ho! , Charles Reade's The Cloister and the Hearth, R. L.
Stevenson's Treasure Island, are typical examples, and the
list might be endlessly extended. Every poet of the nineteenth
century, from Wordsworth to Tennyson and Browning, has left
upon his pages some impression of his travels. From Fielding
to Stevenson one may dip into the novelists almost at random
to find sketches of travel. The first chapter of Guy Mannering is
a vivid picture of a Scottish journey. Tom Jones and Humphrey
Clinker take us along the country roads of England. Vanity Fair
gives a picture of continental travel before the days of railways:
Pickwick is fresh with the more homely humours of the English
roadside and coaching inn. Upon another plane, Charles Lever's
wanderings inspire his pen. Later literature abounds with smaller
books of the same family-fictitious or half-fictitious stories of
trips on foot or bicycle, in canoe or caravan, at home and abroad.
One other reflection occurs. Although the literature of travel
is not the highest kind, and, indeed, cannot be called a distinct
branch, of literature, yet a history of English literature rightly
assigns a space apart to such books, because this kind of writing,
perhaps more than any other, both expresses and influences
national predilections and national character. In view of the
a
## p. 256 (#286) ############################################
256 Literature of Travel, 1700—1900 ([
CH. VII
magnificent achievements and splendid records of other nations
who have preceded or accompanied the British in the fields of
travel and discovery, it would be most inappropriate to attempt
any kind of national comparison. But books of travel and books
inspired by travel have, probably, been more read in Great Britain
than any other books except novels. The educational value of
pleasant travel-books is great. They have provided the substance
of a thousand books for boys; and thus, both directly and in-
directly, have guided and fired the inclinations of many generations
of boys. And every reader, whether boy or man, finds in his
favourite books of travel some image of himself and some hint
towards moulding himself.
## p. 257 (#287) ############################################
CHAPTER VIII
THE LITERATURE OF SCIENCE
A.
PHYSICS AND MATHEMATICS
course.
THE brilliant achievements of British mathematicians, astro-
nomers and physicists under the influence of Isaac Newton
were followed by a long period of comparative inactivity. This
was largely due to the fact that, during a considerable part of the
eighteenth century, members of the British school were, more or less,
out of touch with their continental contemporaries. A free exchange
of views is essential to vigour and, the more varied the outlook
and training of those concerned, the more fruitful is the inter-
The effect of this isolation, moreover, was intensified by
the manner in which English writers strove in their demonstrations
to follow Newtonian forms. If Newton, in his Principia, confined
himself to geometrical proofs, it was because their validity was
unimpeachable; and, since his results were novel, he did not wish
the discussion as to their truth to turn on the methods used to
demonstrate them. But his followers, long after the principles of
the calculus had been accepted, continued to employ geometrical
proofs, whenever it was possible, even when these did not offer
the simplest and most direct way of arriving at the result.
In short, we may say that, in the course of English mathe-
matical science, the last seventy years of the eighteenth century
form a sort of isolated backwater; for this reason, it is unnecessary
here to describe in detail the work of the writers of this period.
We must not, however, fall into the error of thinking that, among
them, there were no men of ability. The investigations of Colin
Maclaurin, of Edinburgh, on attractions, are excellent, and his
treatise on fluxions is, perhaps, the best exposition of that method
of analysis. We may also refer to the work of Thomas Simpson,
of London, on the figure of the earth, tides and various astro-
nomical problems; of John Michell, of Cambridge, who determined
the law of force between magnetic poles, invented the torsion
17
a
E. L. XIV.
CH. VIII.
## p. 258 (#288) ############################################
258 The Literature of Science [CH.
balance and devised the plan of determining the density of the
earth carried out by Cavendish in 1798; of Henry Cavendish",
who discovered the law of attraction in static electricity, introduced
the ideas of electrostatic capacity and specific inductive capacity
and determined the density of the earth by his wellknown
experiments; and of Joseph Priestley! , who also discovered, in-
dependently of others, the law of attraction in electrostatics and
the existence of oxygen ; while, in observational astronomy, we
need only refer to the great achievements of James Bradley and
(Sir) William Herschel. In applications of science, this period
and the early years of the nineteenth century were notable for the
development of the steam-engine. Somewhat earlier, Thomas
Savery and Thomas Newcomen had done much to bring it into
practical use; but modern forms may be said to date from the
improvements introduced by James Watt, Richard Trevithick and
Henry Bell.
With the nineteenth century, a new era in the history of
mathematics and theoretical physics in Great Britain opened.
We shall deal here only with its main features, and, so far as
possible, shall avoid technical details. Unfortunately, limits of
space forbid the introduction of those biographical touches which
would have added to the human interest of the story we have
to tell.
The first thirty or thirty-five years of this period were largely
occupied with work preparatory to the outburst of activity that
characterised the Victorian renascence. Early in the nineteenth
century, the use of analytical methods was introduced in the
Cambridge mathematical curriculum. The advocacy of this change,
originated by Robert Woodhouse, was warmly taken up by George
Peacock, Charles Babbage, (Sir) John Herschel, William Whewell
and (Sir) George Airy. These men worked under the influence of
the great French school, of which Lagrange and Laplace are the
most prominent members, and were hardly affected by their con-
temporaries, such as Gauss, Abel and Jacobi, who were then creating
new branches of pure mathematics. In England, at the beginning
of the century, Cambridge was recognised as the principal mathe-
matical school: all the reformers were residents there, and they
directed their efforts mainly to the introduction of a free use
of analysis in the university course of study. They were
successful ; and, by 1830, the fluxional and geometrical methods
of the eighteenth century had fallen into disuse. The leadership
1 See section B of the present chapter.
## p. 259 (#289) ############################################
Vill] Royal Institution. British Association 259
of Cambridge in this change was undisputed, and the employment
of analytical methods became usual throughout Great Britain.
In these years, a good deal of interesting work in physics and
chemistry was done in London, where the Royal Institution in its
laboratories offered far better opportunities for research than any
similar body in Britain. In connection with this society, we may
mention the work of Thomas Young, whose investigations on wave
motion prepared the way for the acceptance of the undulatory
theory of light, and we may associate with him the names of
(Count) Rumford and (Sir) David Brewster; optics and heat being
the subjects to which their especial attention was directed. At
the same time, John Dalton', in Manchester, was studying the
expansion of gases under varying changes of pressure and
temperature, and the tension of vapours.
At this time, interest in natural philosophy was widely dissemi-
nated, and, in science, as in politics and literature, new ideas were
readily welcomed. Institutes and scientific societies were founded
everywhere, and popular lectures by experts spread broadcast
general, though somewhat vague, information on natural philosophy
and astronomy. The year 1831 is memorable for the foundation
of the British Association for the Advancement of Science. The
intention of its promoters was that the Association should meet
every year for a few days at a provincial town under a distin-
guished president, with the object, partly, of encouraging personal
intercourse between leading men of science and, partly, of pro-
moting interest in scientific work in the various localities where
meetings were held. The meetings led to the regular appointment
of expert committees instructed to report on the progress in various
subjects; these reports have been, and are, of permanent value.
By way of addition to this preliminary statement, we may
also, in passing, mention the History of the Inductive Sciences,
published by Whewell in 1837. It put together in a readable
form the leading facts connected with the history and growth of
science, and, though open to criticism on questions of details-
as was inevitable in the case of an encyclopaedic work of the
kind-it served a useful purpose. Hardly less important was The
Penny Cyclopaedia, issued in twenty-seven volumes in 1833—43
with three supplements.
The most notable physicist at the beginning of the Victorian
period was Michael Faraday), who, in 1831, had begun those
investigations on electricity which have altered our conceptions
1 See section B of the present chapter.
17-2
## p. 260 (#290) ############################################
260
[CH.
The Literature of Science
of the subject, and, by their applications, have revolutionised
industrial science. Faraday had been brought up in humble
circumstances, and his career is interesting as an illustration of
the fact that, in England, no door is closed to genius. In 1812,
after attending some lectures delivered by Sir Humphry Davy,
'he sent notes of them to Davy, asking his assistance to enable him
to study science. The result was that Davy employed him as an
assistant in the chemical laboratory in the Royal Institution.
Here, Faraday's experimental skill soon led to appreciation of his
powers, and he wrote various papers on scientific questions.
Faraday's earliest electrical work related to induced currents,
and depended on his discovery of the fact that, if a wire in the shape
of a closed curve is moved to or from another wire through which
an electric current is flowing, a current is set up in the former
wire which ceases so soon as the motion ceases. The induced
current is caused by and depends on the motion of the one
wire relative to the other. Magnetic effects can be similarly
produced. Faraday went on to explain various phenomena by
the action of the induced currents which he had discovered.
As he pondered on possible explanations of these results, it
occurred to him that all space might be filled by lines of
magnetic force, every line being a closed curve passing through
the magnet to which it belongs ; and he pointed out that the
existence of these lines was suggested by the familiar experi-
ment of the arrangement of iron filings in such lines about a
magnet from whose poles they radiate. According to this view, ,
these induced currents were caused by the closed wire (or any
conductor) being moved across lines of force in its plane of motion,
and, if so, the electromotive force of an induced current would be
proportional to the number of unit lines of magnetic force cut in a
second by the moving wire. Now, the earth itself may be regarded
as a gigantic magnet, and, hence, if a copper wire spin across the
earth's lines of force, we should expect currents to be produced.
This was found to be the case. By these experiments, Faraday
tapped vast and hitherto unknown sources of electricity. The
use of dynamos as a source of mechanical power resulted from
these discoveries.
These investigations were followed by experiments to show
the identity in kind of electrical currents, however produced.
His investigations on electrolysis attracted general attention to
the subject, and led him to the remarkable conclusion that there
is a certain absolute quantity of electricity associated with each
## p. 261 (#291) ############################################
VIII]
Faraday
261
atom of matter. A few years later, in 1845, he discovered another
remarkable series of phenomena dependent on the fact that the
plane of polarisation of light can be rotated by the action of
magnets and electric currents; and, somewhat later, he discovered
and investigated diamagnetic properties in bodies.
The provision of well equipped laboratories is a modern
luxury, and Faraday was exceptionally fortunate in having access
to one. It is difficult to overrate his abilities as an experimental
philosopher; and, though he knew but little mathematics, his
conception of lines of force was essentially mathematical, and
was developed later by Clerk Maxwell and other writers. At the
time, however, it repelled mathematicians accustomed to the
formulae and symbols with which Laplace and Poisson had made
them familiar. It is interesting to see that Faraday, like Newton,
refused to contemplate the possibility of action at a distance, but
sought, rather, to explain the phenomena of attraction by changes
in a continuous medium. He was followed at the Royal Institution
by John Tyndall, whose lectures did much to excite and maintain
general interest in physical questions.
While Faraday was opening new ways of regarding physical
phenomena, the classical methods of Poisson were being applied
with success by James MacCullagh, of Dublin, to problems of
physical optics. In these investigations, MacCullagh, like his
continental contemporaries, elaborated the conception of the
ether as an elastic solid, and, thence, he deduced the laws of re-
flection and refraction ; but, though his work was ingenious, many
of his conclusions were vitiated by his erroneous assumption that
the vibrations of plane polarised light are parallel to the plane of
polarisation. Another physicist of this time whose work has
been of importance was James Prescott Joule, a pupil of Dalton,
who showed that heat and energy were interchangeable in definite
proportions. Mention should also be made of (Sir) Charles
Wheatstone, who, about 1810, brought electric telegraphy into
general use. Wheatstone was a man of wide interests : he early
suggested the use of spectrum analysis for chemical researches
invented stereoscopic instruments and, later, did much useful work
in the construction of dynamos.
This period was rich in inventions whereby science was applied
practically, as, for example, the general employment of steam-
engines for locomotion, the electric telegraph and the introduction
of lighting and heating by gas.
We turn from these practical applications to consider more
## p. 262 (#292) ############################################
262 The Literature of Science [CH.
abstract researches. Faraday was recognised as an exceptional
genius, and time has strengthened the recognition of his claim to
distinction ; but, in general, theoretical physics had, by now,
become so closely connected with mathematics that it seemed
hardly possible for anyone without mathematical knowledge to
make further advances in its problems. This association lasted
well into the twentieth century, and the continuation and extension
of Faraday's work fell into the hands of mathematicians.
Before proceeding to describe the remarkable work of the
school of mathematical physicists who followed Faraday, it will be
convenient to mention the leading writers of this time on pure
mathematics. We may begin by noting the fact that the range
of pure mathematics had, ere this, grown to an extent which
rendered it difficult for any man to master more than a compara-
tively small section of it, and, a fortiori, physicists took up only such
special branches of mathematics as were required for their own
purposes. We should also notice that one of the striking features
of this period has been the largely increased number of students
of mathematical and physical science: hence, to mention only
the leading writers does indirect injustice to others whose work,
though not epoch-making, has been of real importance. With
this caution, we proceed to name a few of those whose researches
have permanently affected the development of mathematics.
In the period on which we are now entering, we find half-a-
dozen mathematicians—De Morgan, Hamilton, Sylvester, Adams,
Cayley and Smith—whose researches will always make it memor-
able. Hamilton and Smith were fastidious writers, and, apart
from the value of their work, it is a pleasure to observe the
artistic manner in which they presented it; but their pupils
were few, and it was only to a select number of scholars that their
writings appealed. The others were more fortunate in being
connected with the great mathematical school of Cambridge.
Their methods are sharply contrasted. De Morgan wrote vi-
vaciously, and largely for non-specialists. Cayley's writings were
precise and methodical, and he always sought to be exhaustive.
Sylvester's papers, like his lectures, were badly constructed,
impetuous and often unfinished ; yet, experience proved them to
be amazingly stimulating. Adams's work was elegant and highly
polished. Modern pure mathematics deals so largely with abstract
and special subjects that it is almost impossible to describe the con-
clusions in a way intelligible to laymen. It will suffice to indicate
the subjects of their principal researches.
## p. 263 (#293) ############################################
vi]
Pure Mathematics
263
Of these mathematicians, Augustus De Morgan was the oldest.
He was educated at Cambridge, but, at that time, office in the
university was conditional on certain declarations of religious
belief. In consequence of this, he moved to London, and there,
through his writings and lectures, exercised wide influence. He
was well read in the philosophy and history of mathematics ; but
it is on the general influence he exerted rather than on dis-
coveries of his own that his reputation rests. With his name
we may associate that of George Boole, of Cork, the creator of
certain branches of symbolic logic, whose mathematical works are
enriched by discussions on the fundamental principles of the
subject. His writings are valuable in themselves, and their pre-
sentment of conclusions is lucid and interesting.
(Sir) William Rowan Hamilton was among the first of a small
but brilliant school of mathematicians connected with Trinity
college, Dublin, where he spent his life. We regard his papers
on optics and dynamics as specially characteristic of his clearness
of exposition: theoretical dynamics being properly treated as a
branch of pure mathematics. He is, however, best known by his
introduction, in 1852, of quaternions as a method of analysis.
Hamilton, followed, later, by authorities so good as P. G. Tait of
Cambridge and Edinburgh, A. Macfarlane of Edinburgh and
Pennsylvania and C. J. Joly of Dublin, asserted that this would
be found to be a potent instrument of research; but, as a matter
of fact, though it lends itself to concise and elegant demonstra-
tions, it is but little used by mathematicians today. In connection
with Dublin, at this time, we must also mention the name of
George Salmon, provost of Trinity college, whose works on ana-
lytical geometry and higher algebra are classical examples of how
advanced text-books should be written, and that of (Sir) Robert
Stawell Ball, first, of Dublin and, later, of Cambridge, who
produced a classical treatise on the theory of screws.
James Joseph Sylvester, like De Morgan, found an academic
life at Cambridge denied him in consequence of his theological
tenets ; but the subsequent abolition of religious tests at the older
universities enabled him, towards the end of his life, to accept
a chair at Oxford. He was a prolific writer; perhaps his favourite
studies were the theory of numbers and higher algebra ; in the
latter subject, he dealt especially with canonical forms, contra-
variants and reciprocants. The lectures that he gave at Baltimore
from 1877 to 1883, did much to stimulate interest in pure
mathematics in America.
7
## p. 264 (#294) ############################################
264
The Literature of Science
[CH.
John Couch Adams was another graduate of Cambridge, and
spent all his life in that university. There are three important
questions in theoretical astronomy, treated as a branch of pure
mathematics, which are especially connected with his name. The
first of these is his discovery, in 1846, of the planet Neptune,
through the disturbance caused by it in the orbit of Uranus ; this
was made independently of, and a few months earlier than, the
similar investigation by Leverrier. This finding of an unsuspected
and unseen planet afforded a striking demonstration of the uni-
versality of gravitation, and excited widespread admiration. The
second of these famous investigations is to be found in Adams's
discussion, published in 1855, of the secular acceleration of
the moon's mean motion—a difficult problem, involving heavy
analytical work and elaborate historical enquiries. The third is
his determination, in 1867, of the orbit of the Leonid shooting
stars.
Arthur Cayley, likewise, spent the bulk of his life at Cambridge,
first as a student and then as a professor. He discussed many
subjects in pure mathematics, his most notable researches dealing
with the general theory of curves and surfaces in analyticalgeometry,
with the theory of invariants in higher algebra, and, in ten classical
memoirs, with binary and ternary forms. He also wrote at length
on elliptic functions, but treated it from Jacobi's point of view;
and, in consequence of Weierstrass's work, much of this is out
of date.
Henry John Stephen Smith, who was educated at the sister
university of Oxford, will be long remembered for his work on the
theory of numbers, especially on linear determinate equations, and
the orders and genera of ternary quadratic forms. He was a
graceful lecturer and writer ; but, while the value of his researches
was recognised, he founded no school. His paper on the repre-
sentation of numbers by sums of four, six, eight, five and seven
squares was the occasion of a curious incident, which illustrates,
incidentally, the widespread ignorance of his work. Fourteen
years after it had been published in the Proceedings of the
Royal Society, the problem, for the single case of five squares, was
proposed by the French Academy as a subject for its grand prize,
open to the world. The problem had, in fact, years before, been
completely solved by Smith, who, to secure the reward had only
to write out his demonstration for the special case proposed.
We have already briefly described Adams's investigations in
mathematical astronomy, and, perhaps, we may here add a word
## p. 265 (#295) ############################################
VII]
Theoretical Physics
265
or two on the researches of (Sir) George Howard Darwin, also of
Cambridge, who investigated the form taken by a rotating viscous
mass of matter, and showed that, in the early history of the solar
system, the moon arose from a portion of the earth thrown off
(when the latter was in a plastic condition) through its increasing
velocity of rotation. Later, he demonstrated that the moons of
the other planets could not have originated in the same way. He
wrote at length on the theory of tides. He also worked at the
problem of three bodies, investigating, by lengthy arithmetical
methods, possible stable forms of periodic orbits of one body,
moving under the attraction of two other bodies.
With observational and practical astronomy we are not here
concerned; but we may add that the results of the astronomical
discoveries of the Victorian period were made familiar to the
English speaking world by the popular treatises and lectures of
Sir Robert S. Ball whom we have already mentioned, and by
various works by Miss A. M. Clerke.
Mention may here be made, also, of two great teachers of the
Victorian age, to wit, William Hopkins, and Edward John Routh,
under whom many generations of Cambridge mathematicians were
educated, and to whom the predominance in Britain, throughout
the period here treated, of the mathematical school of that university
is largely due. Of more recent English writers on pure mathematics,
some have devoted themselves to higher analysis, especially
differential equations, differential geometry and the theory of
functions ; others have followed continental initiative in discuss-
}
ing the fundamental principles and philosophy of mathematics.
We return to the subject of theoretical physics.
It was the
good fortune of the Cambridge school to produce, in the Victorian
period, some of the greatest physicists of the century. The
university course for a degree, at that time, involved a study of
the elements of nearly all the branches of mathematics then read;
and, thus, its graduates were exceptionally well equipped for
discussing physical problems from the mathematical side. Among
these physicists, we here mention briefly the work of George Green,
(Sir) George Stokes, (Sir) William Thomson afterwards lord Kelvin,
and Clerk Maxwell. To their credit, be it said, they all treated
symbols and formulae as servants and not as ends in themselves.
George Green was a self-educated man, who came to Cambridge
in middle life and took his degree in 1837, unfortunately for science
dying four years later. In 1828, he introduced the idea of the
potential, representing the work which must be done to move a
## p. 266 (#296) ############################################
266 The Literature of Science [CH.
unit of mass from infinity to its position. In this memoir is
established the celebrated formula, connecting surface and volume
integrals, which forms a fundamental proposition in the theory of
attractions. Green wrote on various physical questions, notably
on the motion of waves in a canal, and the deduction of the
geometrical laws of sound and light from the undulatory theory.
In these writings, he showed remarkable physical insight in the
applications of his analysis. His memoirs on the propagation of
light in a crystalline medium, published in 1839, rest on the
assumption that the ether in a crystal resembles an elastic solid
unequally pressed in different directions by unmoved ponderable
matter—a conception which, later, was to lead to remarkable
developments. Few writings have been more fruitful than those
of Green. They led MacCullagh and Cauchy to revise their theories
of optics, and they profoundly impressed Stokes and Kelvin, whose
work we now proceed to describe.
(Sir) George Gabriel Stokes spent his life at Cambridge, where
he held the Lucasian chair for over half a century. Through his
long tenure of the secretaryship of the Royal society, he acted as
the friend and guide of innumerable young authors, for, by virtue
of his office, he saw the manuscripts of all papers on mathematics
and physics, and freely placed at the disposal of the writers his
unrivalled knowledge of physics and mathematics : thus, a con-
siderable proportion of his work appears under the names of other
writers. He began his scientific career under the influence of
Green's writings. It is difficult to describe his researches in
general terms. The most important of them are concerned with
optics, hydrodynamics and geodesy. In optics, he was mainly
responsible for the explanation of fluorescence, and only just
missed being the first to propound the true explanation of
Fraunhofer's lines; he subjected diffraction to mathematical
analysis ; in hydrodynamics, we owe to him the modern theory
of viscous fluids, and he wrote on the properties and constitution
of the ether. His work in pure mathematics, especially on the
convergence of series, was also of importance. Stokes was an
excellent man of affairs-he sat for a time in the house of
commons—but his gift of silence prevented his exercising among
strangers the full influence which his abilities deserved. He was
the intimate friend of Kelvin and Maxwell, and to his deliberate
judgment on scientific matters Kelvin always yielded.
(Sir) William Thomson, later raised to the peerage under the
title baron Kelvin, was another graduate of Cambridge of this
## p. 267 (#297) ############################################
VIII]
Kelvin
267
period. (To avoid the confusion of the use of two names we will
here refer to him as Kelvin. ) In 1846, the year following on
his first degree, he accepted a professorial chair at Glasgow, but
he always kept in touch with his mathematical friends in Cambridge.
Probably, he exercised a wider influence in the world at large
than any of his scientific contemporaries; but his interests were so
catholic that it is not easy to give any connected account of them.
He possessed an almost intuitive power of realising fundamental
principles. Throughout his life, ideas seem to have come to him
so rapidly as to give him insufficient time for their effective
development; hence, the student will search in vain among his
papers for complete and systematic expositions of his discoveries.
He began his career under the influence of Fourier, Green and
Faraday. Electricity was his favourite subject of research. The
writer of this sketch has heard him illustrate the progress in this
subject by the fact that, in his early life, he was accustomed to
explain his conclusions in it by analogies drawn from the theory
of heat; but, before he died, problems in heat were commonly
illustrated by analogous questions in electricity. Kelvin wrote
at length on the subject of electromagnetic fields, put forward
numerous suggestions about the constitution of ether and matter,
and laid the foundation for a scientific system of measurement of
electrical quantities. · Hydrodynamics, elasticity and thermo-
dynamics were other subjects on which he wrote, and his papers
on energy and entropy were of far-reaching importance. We
cannot leave Kelvin's work without mentioning the appearance,
n 1867, of the treatise on natural philosophy by himself and his
friend Peter Guthrie Tait, of Cambridge and Edinburgh. In spite
of Tait's collaboration, this book presents an unfinished aspect;
but it is suggestive, and it widely affected modes of physical
thought throughout Europe.
The theory of signalling by cable originated in a correspondence
between Kelvin and Stokes in 1854, and was elaborated by Kelvin
and Kirchhoff, while to the former are largely due the practical
applications of it. The earliest successful submarine cable laid
between England and the continent dates only from 1851. Owing
to the large capacity of the cable and the soakage into the
insulating material, powerful currents had to be used before
sensible effects could be obtained, and these difficulties increased
with the length of the cable. Accordingly, when, in 1857, a cable
was laid to America, the operators deemed it necessary to use
electricity of a high tension, with the result that the insulation
## p. 268 (#298) ############################################
268 The Literature of Science [Ch.
a
was ruptured. Subsequently, Kelvin was given a free hand in
arranging a system for use with a later cable. He evaded the old
difficulties by developing extreme sensitiveness in the receiving
instruments and applied the methods of Gauss and Weber for
indicating the minute motions of the oscillating needle by the
reflection of a ray of light from it, in effect employing a long
non-material pointer. In 1870, he substituted for this method
a syphon-recorder which printed the message ; and this instrument
is still sometimes used. He held that the transmission of signals
along the wire of a submarine cable was due to an actual dis-
turbance in the wire : whereas, according to the modern theory,
propounded by Maxwell, the function of the wire is merely to
guide the disturbance resident in the surrounding dielectric.
Kelvin was a keen yachtsman, and was thus led to take up the
problem of compasses ; he also bore an active part in the develop-
ment of electrical engineering. He was the owner of several
patents connected with these practical applications of science.
We have next to mention one whose work has had so important
an influence on the subsequent growth of the subject as to make
it the beginning of a new epoch. This was James Clerk Maxwell
-the most modest of men-another member of the Cambridge
school, who, for the last eight years of his life, occupied
in the university the then newly created chair of experimental
physics.
Since the time of Descartes, natural philosophers had never
ceased to speculate on the processes by which gravity, light and
electricity are transmitted through space. So far as electricity
is concerned, the idea of lines of force in a continuous medium is
due to Faraday. Kelvin, as a young man, had suggested that
electric force might be transmitted through a medium, somewhat
as elastic displacements are transmitted through an elastic solid.
This idea was taken up by Maxwell, who, in 1856, elaborated the
analogies offered by the flow of a liquid, and, five years later,
devised a mechanical model of electromagnetic action. He now
brought forward a series of arguments to show that an electric
current was a phenomenon of translation, magnetism one of
rotation and the electrostatic state one of strain of the ether.
These conclusions led him to assert that light consists of trans-
verse waves of the same medium as that required for the
explanation of electric and magnetic phenomena. On this theory,
all currents are closed; magnetic energy is the kinetic energy
of the ether, and electric energy the energy of 'strain of the ether.
## p. 269 (#299) ############################################
VIII)
Maxwell's Electromagnetic Theory 269
These views were presented, as a whole, in 1864. Further ex-
tensions and developments of the theory followed, and the whole
was set out in his treatise published in 1873. This celebrated
work is far from easy to read, and the exposition is not
systematic, but it may be said that the fundamental ideas are now
universally accepted, and most of the work of his successors has
been built on the foundation here laid. The theory was based on
Faraday's ideas; but it required a trained mathematician to give
the final form to his conceptions and to deduce their consequences.
Hence, the theory is properly associated with Maxwell's name.
Maxwell, also, took a considerable part in framing a standard
system of electrical measurements. He contributed largely to the
kinetic theory of gases, and, incidentally, to theories on the con-
stitution of matter.
A large part of the history of mathematical physics during the
last quarter of the nineteenth century consists of the completion
and extension of Maxwell's electromagnetic theory. No incon-
siderable part of this is due to his successors at Cambridge, and to
describe recent researches in physics without mentioning the names
of lord Rayleigh, Sir Joseph John Thomson and Sir Joseph Larmor
is almost impossible; here, however, we must content ourselves
with a very brief account of the general line of investigation
followed in the last part of the period covered by the present
section.
It has already been pointed out that Maxwell's exposition of
his electromagnetic theory of light was neither systematic nor
complete. A curious omission in it was the absence of any
explanation of reflection and refraction ; this was supplied by
Helmholtz. The problem of the effects produced by the translation
of electric charges, raised by the same investigator, was solved by
the researches of the present Cavendish professor at Cambridge,
George Francis FitzGerald of Dublin, and others : in the mathe-
matical development of the theory, which now proceeded apace,
they, again, took a prominent part. In 1883, FitzGerald explained
a system of magnetic oscillators by which radiant energy could
be obtained from electrical sources, thus confirming Maxwell's
theoretical conclusion that light was an electromagnetic phe-
Some of Maxwell's assumptions on which he had
based his theory still remained unconfirmed; but, a year or two
later, the theory was placed on a firmer experimental basis by
Hertz. The results, incidentally, led to the introduction of wire-
less telegraphy.
nomenon.
## p. 270 (#300) ############################################
270 The Literature of Science [CH.
The question of the conduction of electric discharges through
liquids and gases had been raised by Faraday. It was now taken
up seriously, and various types of rays, cathode rays, Röntgen
rays, etc. , were discovered. These researches led to new views on
the constitution of matter. The investigations began with a
theory of electrons, and, finally, led to the view that every so-
called atom is formed by a combination of two elements in varying
proportions, and that, possibly, these two elements are to be
identified with forms of electricity-one of the most far-reaching
hypotheses propounded in recent times.
The efforts to extend the theory of the electromagnetic field to
cases where heavy masses are in motion introduces the difficult
question as to whether the ether round and in bodies is affected
by their motion, and to this theory of relativity much attention
is now being paid.
One of the striking features of the Victorian period has been
the equipment of large laboratories where experiments can be
carried out by students with an accuracy wholly impossible in
former days. Two of the earliest of these were built at Oxford
and Cambridge, the former known as the Clarendon laboratory
in 1872, the latter, known as the Cavendish laboratory, being
the gift of the seventh duke of Devonshire. In the latter,
Clerk Maxwell taught and has been succeeded by professors
not less distinguished. The existence of such laboratories in
seats of learning has profoundly affected the teaching of the
subject by training large numbers of competent observers, besides
calling forth in ever widening circles an intelligent interest in
physical studies.
It is not, we think, too much to say that the work in physics of
the Victorian period has completely revolutionised the subject,
and, both on its theoretical and practical sides, far exceeds in value
that previously done in any period of similar extent. The theory
of gravitation was the great achievement of the Newtonian school.
In the following century, physical optics and, later, the nature of
ether attracted most attention from philosophers, while practical
men developed the steam-engine and studied the theory of heat.
The Victorian age has seen electricity raised to the rank of an all-
embracing science, and applied to innumerable industrial uses,
power-engines, lighting, heating, telegraphy, telephones. Other
important scientific and industrial applications relate to photo-
graphy and spectrum analysis ; the development of the turbine-
engine; the invention of the internal combustion-engine, with its
## p. 271 (#301) ############################################
VIII]
Chemistry
271
numerous uses in transport on land and water; the introduction
of submarine boats, and heavier-than-air flying-machines ; and
the use of wireless telegraphy. In this chapter, however, bare
reference to these practical applications must suffice.
B. CHEMISTRY
Chemistry has always busied itself with the changes of
material things. By working in metals and precious stones, by
making colours, by producing things used by artists to give
delight to themselves and others, by fashioning natural materials
into things useful to men, by concocting potions which had strange
effects on the bodies and minds of those who swallowed them, by
doing these things and things like these, chemists slowly amassed
much knowledge, knowledge, however, which was fragmentary and
disconnected. The strange changes which chemists discovered
impelled the more ardent and adventurous among them to dream
of the possibility of finding a universal medicine which should put
an end to disease and suffering and enable the adept to bring all
imperfect things to a state of perfection. The history of alchemy
is the history of a particular branch of the universal quest, the
quest of the unchanging.
In the later years of the eighteenth century, between 1770 and
1790, chemistry passed, at a bound, from being an empirical art to
becoming a science. The man who made the great transformation
was Antoine Laurent Lavoisier. With the work of the master we
are not concerned here.
From the time of Lavoisier to our own day, chemistry has
progressed, in the main, along four lines. For some years, chemists
concentrated their attention on one definite class of material
changes, the changes which happen when substances are burned
in the air. The knowledge which was gained of the changes of
composition and of properties during combustion incited and
guided chemists to a searching examination of the distinctive
properties of many different substances, and this examination
brought about the clarifying of the conception of definite kinds
of matter, and the application of this conception to the opening of
many paths of chemical enquiry. While these advances were being
made, a quiet member of the Society of Friends presented chemistry
with a marvellously delicate and penetrative instrument for further-
ing accurate knowledge of material changes. John Dalton made
## p. 272 (#302) ############################################
272
The Literature of Science [CH.
what seemed a small addition to the Greek atomic theory, an
addition which changed an interesting speculation into a scientific
theory. As the century went on, chemists began to elucidate
the connections between chemical events and physical phenomena.
The science of physical chemistry began.
Among those who investigated the phenomena of combustion
in the eighteenth, and early nineteenth, century, Priestley and
Cavendish are pre-eminent. Black was the first chemist to
make an accurate, quantitative examination of a particular,
limited, chemical change, and, by so doing, to give clearness to
the expression 'a homogeneous substance. ' The atomic theory
was Dalton's gift to science. From the many chemists who
amplified the work of Dalton, and used the conceptions of atom
and molecule to connect and explain new classes of chemical facts,
Williamson and Frankland may be selected as the representatives.
As workers in the borderland between chemistry and physics,
Graham and Faraday are specially to be remembered. The in-
vestigations of Davy touched and illuminated every side of
chemical progress.
Besides these men, who greatly enriched and advanced the
science of chemistry in the period under review, there were
many workers whose contributions cannot be considered here,
References are given in the bibliography to the writings of some
of them.
Joseph Priestley was a man of many gifts and a very versatile
mind. When a youth at an academy, he tells us that he 'saw
reason to embrace what is generally called the heterodox side of
almost every question. When about twenty-eight years of age,
he taught, in a school at Warrington, languages (he had a great
natural gift of tongues), oratory and criticism, elocution, logic,
natural phenomena, civil law and anatomy.
In the seventies of the eighteenth century, Priestley turned his
attention to different kinds of airs. He obtained and partially
examined many gases, but rarely troubled about separating them
completely from impurities. In August 1774, Priestley obtained a
large lens with which he concentrated the sun's rays on whatever
substance happened to come to his hand, with the object of finding
what air could be extracted from it. When he thus heated mer-
curius calcinatus per se (now called oxide of mercury), he obtained
an air in which a candle burned with a remarkably vigorous
flame. ' This result, he says, 'surprised me more than I can well
express. ' The new air was subjected to many tests; it always
6
## p. 273 (#303) ############################################
VIII] Priestley's Work on Gases
273
behaved in a very unexpected manner. He placed a mouse in his
new air; the mouse remained lively, and the air did not become
noxious. ' The results of other experiments caused Priestley
to lie awake through the night ‘in utter astonishment. ' At last,
he concluded that the new air was 'between four and five times as
good as common air. He regarded the new air as a very superior
kind of common air.
Priestley thought alchemically, not as a chemist. To the
alchemist, the properties of things were external wrappings which
might be removed from one thing and put round another, without
affecting the essential substance of either thing, which substance
it was the business of properties to hide from the uninitiated.
Priestley thought of different airs as identical, or nearly identical,
in substance, and only apparently different because of superficial
differences in the mantles, the properties, by which the essential
substance was concealed. When he obtained the air from burnt
mercury, he thought he had removed from common air something
which made it ‘noxious, vitiated, depraved, corrupt. ' He had not
learnt, what Black’s experiments, made twenty years before 1774,
might have taught him, that each particular, material thing is
known only by its properties. Priestley's forced explanation of the
facts which he himself discovered helped to convince investigators
that the notion of identity of substance hidden under differences
of properties is a great hindrance to the acquirement of accurate
knowledge of natural events.
Priestley could not get over his astonishment at the behaviour
of the new air. In science, one does well to be astonished; but,
to astonishment one must add investigation, to investigation,
reasoning, and, to reasoning, more investigation. Stopping at
astonishment, Priestley made his facts square with the theory that
dominated him, the theory of phlogiston. The phlogisteans taught
that something, which they had named phlogiston, the principle of
fire, rushes out of a burning substance as it burns. Phlogiston
was never captured. Priestley held that the elusive phlogiston is
a great corrupter of your airs or gases. He supposed that he had
deprived common air of this depraving principle; he named his
new gas dephlogisticated air. He invented many very ingenious
hypotheses to account for facts observed by himself. Had he
made a few accurate quantitative experiments, he might have
broken the toils of his favourite theory.
The French chemist Lavoisier saw the importance of Priestley's
discovery of dephlogisticated air, and, by a series of rigidly
18
E. L. XIV.
CH. VIII.
## p. 274 (#304) ############################################
274 The Literature of Science [ch.
>
quantitative experiments with tin and mercury, proved that, when
a substance burns in air, it combines with a constituent of the air,
which air-constituent is the gas prepared by Priestley. Lavoisier
called this gas oxygen, because many of its compounds are
acids.
Priestley's insatiable curiosity, his mental alertness, his im.
patience of details, were required for the advancement of chemistry,
no less than the passionless determination and the scrupulous
accuracy of Cavendish.
Henry Cavendish, of Peterhouse, was bred in the theory of
phlogiston, as Priestley was, and remained faithful to that theory,
as Priestley did. He thought of many airs, or gases, as more or less
phlogisticated forms of a few particular substances. Cavendish
described the explosion of a mixture of common air and inflammable
air (obtained by the action of acids on zinc) as one of the ways of
phlogisticating air. This process is accompanied by a decrease in
the volume of the interacting gases. Cavendish tried to discover
the cause of this decrease. He exploded accurately measured
volumes of dephlogisticated air (oxygen) and inflammable air
(hydrogen), and found that water was the sole product of the
change when dephlogisticated air was mixed with twice its volume
of inflammable air. The explanation which Cavendish gave of
this fundamentally important fact was confused and vague, because
he insisted on making the facts uphold the phlogistic theory.
Without knowing exactly what he had done, Cavendish had
determined the quantitative volumetric composition of water.
When the phlogistic theory had been swept away, the very
great importance of the accurate work of Cavendish became
manifest.
Joseph Black graduated as doctor of medicine in the university
of Edinburgh in 1755, presenting a thesis entitled Magnesia
alba, Quicklime, and other alcaline substances. That thesis is
probably the earliest example of a genuinely scientific chemical
investigation. Black proved that mild magnesia (now called
magnesium carbonate) loses weight when it is calcined; he deter-
mined the loss of weight; he proved that the solid substance
which remains after calcination has properties of its own which
distinguish it from mild magnesia; he showed that, during calci-
nation, an air, or gas, is given off, different from any air, or gas,
then known ; he examined, accurately, the properties of this gas,
which he called fixed air; and he reproduced the original quantity
of mild magnesia by dissolving the calcined magnesia in acid, and
>
## p. 275 (#305) ############################################
VIII) Black and Cavendish
275
adding fixed alkali (now called potassium carbonate), a substance
which he proved to give off fixed air when it is calcined. By his
experiments, Black proved mild magnesia to be composed of fixed
air united with calcined magnesia, and showed that each of these
three substances is a particular and definite kind of matter, dis-
tinguished from all other kinds of matter by constant qualities.
He also proved that the change which happens when chalk is
burned is exactly similar to the calcining of mild magnesia ; fixed
air is driven out of the chalk, and burnt lime—a perfectly
definite homogeneous substance-remains.
The work of Black prepared the way for the penetrative, ex-
perimental analysis of the phenomena of combustion ; it taught
chemists to use accurately observed properties of bodies as the
only means of distinguishing one body from another; it showed
that, if chemical investigation is to produce results of permanent
value, it must be quantitative; incidentally, by isolating and
examining fixed air, it began a new branch of chemistry, the study
of the changes of composition and properties which happen when
homogeneous gases interact.
Black and Cavendish were painstaking, methodical, un-
emotional, eminently clear-headed. Priestley was flighty, fitting
from one thing to another in his laboratory, always curious, never
working out his discoveries, unable to think chemically outside of
the theory which dominated him. Black, Cavendish and Priestley
greatly advanced the science of chemistry.
So long as chemists formed vague generalisations founded on
introspective speculations, they made little progress. It was by
concentrating their attention on a few limited occurrences, and
accurately examining these by quantitative experiments, that
chemists gradually gained clear conceptions which could be
directly used in the investigation of more complicated chemical
changes. “True genius,' Coleridge said, 'begins by generalising
and condensing; it ends in realising and expanding. ' The vague
generalising of the alchemists was followed by the condensing
work of Black and Cavendish, and by the suggestive discoveries of
Priestley. The time was approaching for realising and expanding.
In 1808, a small book appeared, entitled A new system of
Chemical Philosophy, Part I, by John Dalton. The influence of
that book on the development of chemistry, and of physics also,
has been very great.
Dalton delivered a lecture in Manchester, in 1803, wherein he
said 'An enquiry into the relative weights of the ultimate particles
18-2
## p. 276 (#306) ############################################
276
The Literature of Science [CH.
of bodies is a subject, so far as I know, entirely new; I have lately
been prosecuting this enquiry with remarkable success. ' Many of
Dalton's predecessors, both chemists and physicists, had used, in a
vague and general manner, the Greek conception of the atomic
structure of matter. Dalton showed how the relative weights of
atoms can be determined. By doing that, he brought down the
atomic theory to the solid earth, and made it a bold, suggestive,
stimulating guide ready for the use of chemists and physicists.
Dalton was not a great experimenter; he generally used the
results of other chemists' experiments. He was a scientific thinker,
characterised by boldness and caution. Dalton assumed, as
Lucretius had done long before him, that matter has a grained
structure ; that all the ultimate particles of each particular
homogeneous substance are identical, and differ in properties,
one of which is their weight, from the particles of all other
definite substances; he also assumed that the mechanism of
chemical changes, that is, changes wherein homogeneous substances
are produced different from those present when the changes began,
is the coalescence of atoms of different kinds to form new sorts of
atoms.
In order to find the relative weights of atoms, Dalton argued
as follows: Analyses and syntheses of water show that eight
grains of oxygen unite with one grain of hydrogen to form water.
If this change is the union of atoms of oxygen with atoms of
hydrogen, to form atoms of water, and if all the atoms of each
one of these three homogeneous substances are identical in weight
and other properties, it follows that an atom of oxygen is eight
times heavier than an atom of hydrogen. If we take the atomic
weight of hydrogen as unity—because hydrogen is lighter than
any other known substance—then the atomic weight of oxygen is
eight, and the atomic weight of water is nine.
In arriving at the conclusion that the atomic weight of oxygen
is eight, if the atomic weight of hydrogen is one, Dalton made the
assumption that a single atom of oxygen unites with one atom of
hydrogen to form an atom of water. He made this assumption
because it was simpler than any other. Had he chosen to suppose
that two atoms of hydrogen unite with one atom of oxygen, he
must have assigned to oxygen the atomic weight sixteen, and to
water the atomic weight eighteen.
To make Dalton's method perfectly general, and quite conclusive
in its results, it was necessary to find means for fixing the relative
weights of the atoms formed by the union of other, simpler, atoms;
## p. 277 (#307) ############################################
VII] The Atomic Theory. Dalton and Others 277
it was also necessary to find means of determining the number of
atoms of each kind which unite to form a more complex atom.
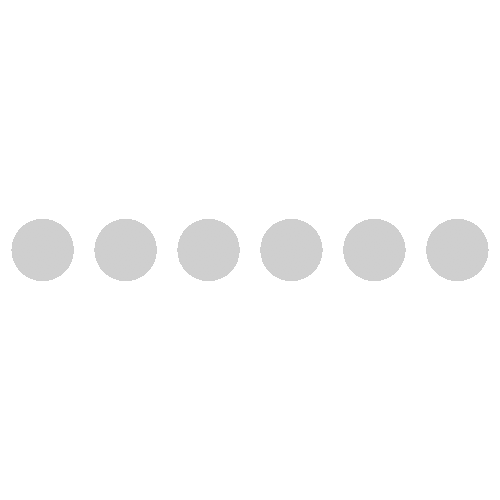