In 1852, Edward Frankland
applied the notion of equivalency to the atoms of elements, that
is, homogeneous substances which have not been separated into
unlike parts.
applied the notion of equivalency to the atoms of elements, that
is, homogeneous substances which have not been separated into
unlike parts.
Cambridge History of English Literature - 1908 - v14
Green wrote on various physical questions, notably
on the motion of waves in a canal, and the deduction of the
geometrical laws of sound and light from the undulatory theory.
In these writings, he showed remarkable physical insight in the
applications of his analysis. His memoirs on the propagation of
light in a crystalline medium, published in 1839, rest on the
assumption that the ether in a crystal resembles an elastic solid
unequally pressed in different directions by unmoved ponderable
matter—a conception which, later, was to lead to remarkable
developments. Few writings have been more fruitful than those
of Green. They led MacCullagh and Cauchy to revise their theories
of optics, and they profoundly impressed Stokes and Kelvin, whose
work we now proceed to describe.
(Sir) George Gabriel Stokes spent his life at Cambridge, where
he held the Lucasian chair for over half a century. Through his
long tenure of the secretaryship of the Royal society, he acted as
the friend and guide of innumerable young authors, for, by virtue
of his office, he saw the manuscripts of all papers on mathematics
and physics, and freely placed at the disposal of the writers his
unrivalled knowledge of physics and mathematics : thus, a con-
siderable proportion of his work appears under the names of other
writers. He began his scientific career under the influence of
Green's writings. It is difficult to describe his researches in
general terms. The most important of them are concerned with
optics, hydrodynamics and geodesy. In optics, he was mainly
responsible for the explanation of fluorescence, and only just
missed being the first to propound the true explanation of
Fraunhofer's lines; he subjected diffraction to mathematical
analysis ; in hydrodynamics, we owe to him the modern theory
of viscous fluids, and he wrote on the properties and constitution
of the ether. His work in pure mathematics, especially on the
convergence of series, was also of importance. Stokes was an
excellent man of affairs-he sat for a time in the house of
commons—but his gift of silence prevented his exercising among
strangers the full influence which his abilities deserved. He was
the intimate friend of Kelvin and Maxwell, and to his deliberate
judgment on scientific matters Kelvin always yielded.
(Sir) William Thomson, later raised to the peerage under the
title baron Kelvin, was another graduate of Cambridge of this
## p. 267 (#297) ############################################
VIII]
Kelvin
267
period. (To avoid the confusion of the use of two names we will
here refer to him as Kelvin. ) In 1846, the year following on
his first degree, he accepted a professorial chair at Glasgow, but
he always kept in touch with his mathematical friends in Cambridge.
Probably, he exercised a wider influence in the world at large
than any of his scientific contemporaries; but his interests were so
catholic that it is not easy to give any connected account of them.
He possessed an almost intuitive power of realising fundamental
principles. Throughout his life, ideas seem to have come to him
so rapidly as to give him insufficient time for their effective
development; hence, the student will search in vain among his
papers for complete and systematic expositions of his discoveries.
He began his career under the influence of Fourier, Green and
Faraday. Electricity was his favourite subject of research. The
writer of this sketch has heard him illustrate the progress in this
subject by the fact that, in his early life, he was accustomed to
explain his conclusions in it by analogies drawn from the theory
of heat; but, before he died, problems in heat were commonly
illustrated by analogous questions in electricity. Kelvin wrote
at length on the subject of electromagnetic fields, put forward
numerous suggestions about the constitution of ether and matter,
and laid the foundation for a scientific system of measurement of
electrical quantities. · Hydrodynamics, elasticity and thermo-
dynamics were other subjects on which he wrote, and his papers
on energy and entropy were of far-reaching importance. We
cannot leave Kelvin's work without mentioning the appearance,
n 1867, of the treatise on natural philosophy by himself and his
friend Peter Guthrie Tait, of Cambridge and Edinburgh. In spite
of Tait's collaboration, this book presents an unfinished aspect;
but it is suggestive, and it widely affected modes of physical
thought throughout Europe.
The theory of signalling by cable originated in a correspondence
between Kelvin and Stokes in 1854, and was elaborated by Kelvin
and Kirchhoff, while to the former are largely due the practical
applications of it. The earliest successful submarine cable laid
between England and the continent dates only from 1851. Owing
to the large capacity of the cable and the soakage into the
insulating material, powerful currents had to be used before
sensible effects could be obtained, and these difficulties increased
with the length of the cable. Accordingly, when, in 1857, a cable
was laid to America, the operators deemed it necessary to use
electricity of a high tension, with the result that the insulation
## p. 268 (#298) ############################################
268 The Literature of Science [Ch.
a
was ruptured. Subsequently, Kelvin was given a free hand in
arranging a system for use with a later cable. He evaded the old
difficulties by developing extreme sensitiveness in the receiving
instruments and applied the methods of Gauss and Weber for
indicating the minute motions of the oscillating needle by the
reflection of a ray of light from it, in effect employing a long
non-material pointer. In 1870, he substituted for this method
a syphon-recorder which printed the message ; and this instrument
is still sometimes used. He held that the transmission of signals
along the wire of a submarine cable was due to an actual dis-
turbance in the wire : whereas, according to the modern theory,
propounded by Maxwell, the function of the wire is merely to
guide the disturbance resident in the surrounding dielectric.
Kelvin was a keen yachtsman, and was thus led to take up the
problem of compasses ; he also bore an active part in the develop-
ment of electrical engineering. He was the owner of several
patents connected with these practical applications of science.
We have next to mention one whose work has had so important
an influence on the subsequent growth of the subject as to make
it the beginning of a new epoch. This was James Clerk Maxwell
-the most modest of men-another member of the Cambridge
school, who, for the last eight years of his life, occupied
in the university the then newly created chair of experimental
physics.
Since the time of Descartes, natural philosophers had never
ceased to speculate on the processes by which gravity, light and
electricity are transmitted through space. So far as electricity
is concerned, the idea of lines of force in a continuous medium is
due to Faraday. Kelvin, as a young man, had suggested that
electric force might be transmitted through a medium, somewhat
as elastic displacements are transmitted through an elastic solid.
This idea was taken up by Maxwell, who, in 1856, elaborated the
analogies offered by the flow of a liquid, and, five years later,
devised a mechanical model of electromagnetic action. He now
brought forward a series of arguments to show that an electric
current was a phenomenon of translation, magnetism one of
rotation and the electrostatic state one of strain of the ether.
These conclusions led him to assert that light consists of trans-
verse waves of the same medium as that required for the
explanation of electric and magnetic phenomena. On this theory,
all currents are closed; magnetic energy is the kinetic energy
of the ether, and electric energy the energy of 'strain of the ether.
## p. 269 (#299) ############################################
VIII)
Maxwell's Electromagnetic Theory 269
These views were presented, as a whole, in 1864. Further ex-
tensions and developments of the theory followed, and the whole
was set out in his treatise published in 1873. This celebrated
work is far from easy to read, and the exposition is not
systematic, but it may be said that the fundamental ideas are now
universally accepted, and most of the work of his successors has
been built on the foundation here laid. The theory was based on
Faraday's ideas; but it required a trained mathematician to give
the final form to his conceptions and to deduce their consequences.
Hence, the theory is properly associated with Maxwell's name.
Maxwell, also, took a considerable part in framing a standard
system of electrical measurements. He contributed largely to the
kinetic theory of gases, and, incidentally, to theories on the con-
stitution of matter.
A large part of the history of mathematical physics during the
last quarter of the nineteenth century consists of the completion
and extension of Maxwell's electromagnetic theory. No incon-
siderable part of this is due to his successors at Cambridge, and to
describe recent researches in physics without mentioning the names
of lord Rayleigh, Sir Joseph John Thomson and Sir Joseph Larmor
is almost impossible; here, however, we must content ourselves
with a very brief account of the general line of investigation
followed in the last part of the period covered by the present
section.
It has already been pointed out that Maxwell's exposition of
his electromagnetic theory of light was neither systematic nor
complete. A curious omission in it was the absence of any
explanation of reflection and refraction ; this was supplied by
Helmholtz. The problem of the effects produced by the translation
of electric charges, raised by the same investigator, was solved by
the researches of the present Cavendish professor at Cambridge,
George Francis FitzGerald of Dublin, and others : in the mathe-
matical development of the theory, which now proceeded apace,
they, again, took a prominent part. In 1883, FitzGerald explained
a system of magnetic oscillators by which radiant energy could
be obtained from electrical sources, thus confirming Maxwell's
theoretical conclusion that light was an electromagnetic phe-
Some of Maxwell's assumptions on which he had
based his theory still remained unconfirmed; but, a year or two
later, the theory was placed on a firmer experimental basis by
Hertz. The results, incidentally, led to the introduction of wire-
less telegraphy.
nomenon.
## p. 270 (#300) ############################################
270 The Literature of Science [CH.
The question of the conduction of electric discharges through
liquids and gases had been raised by Faraday. It was now taken
up seriously, and various types of rays, cathode rays, Röntgen
rays, etc. , were discovered. These researches led to new views on
the constitution of matter. The investigations began with a
theory of electrons, and, finally, led to the view that every so-
called atom is formed by a combination of two elements in varying
proportions, and that, possibly, these two elements are to be
identified with forms of electricity-one of the most far-reaching
hypotheses propounded in recent times.
The efforts to extend the theory of the electromagnetic field to
cases where heavy masses are in motion introduces the difficult
question as to whether the ether round and in bodies is affected
by their motion, and to this theory of relativity much attention
is now being paid.
One of the striking features of the Victorian period has been
the equipment of large laboratories where experiments can be
carried out by students with an accuracy wholly impossible in
former days. Two of the earliest of these were built at Oxford
and Cambridge, the former known as the Clarendon laboratory
in 1872, the latter, known as the Cavendish laboratory, being
the gift of the seventh duke of Devonshire. In the latter,
Clerk Maxwell taught and has been succeeded by professors
not less distinguished. The existence of such laboratories in
seats of learning has profoundly affected the teaching of the
subject by training large numbers of competent observers, besides
calling forth in ever widening circles an intelligent interest in
physical studies.
It is not, we think, too much to say that the work in physics of
the Victorian period has completely revolutionised the subject,
and, both on its theoretical and practical sides, far exceeds in value
that previously done in any period of similar extent. The theory
of gravitation was the great achievement of the Newtonian school.
In the following century, physical optics and, later, the nature of
ether attracted most attention from philosophers, while practical
men developed the steam-engine and studied the theory of heat.
The Victorian age has seen electricity raised to the rank of an all-
embracing science, and applied to innumerable industrial uses,
power-engines, lighting, heating, telegraphy, telephones. Other
important scientific and industrial applications relate to photo-
graphy and spectrum analysis ; the development of the turbine-
engine; the invention of the internal combustion-engine, with its
## p. 271 (#301) ############################################
VIII]
Chemistry
271
numerous uses in transport on land and water; the introduction
of submarine boats, and heavier-than-air flying-machines ; and
the use of wireless telegraphy. In this chapter, however, bare
reference to these practical applications must suffice.
B. CHEMISTRY
Chemistry has always busied itself with the changes of
material things. By working in metals and precious stones, by
making colours, by producing things used by artists to give
delight to themselves and others, by fashioning natural materials
into things useful to men, by concocting potions which had strange
effects on the bodies and minds of those who swallowed them, by
doing these things and things like these, chemists slowly amassed
much knowledge, knowledge, however, which was fragmentary and
disconnected. The strange changes which chemists discovered
impelled the more ardent and adventurous among them to dream
of the possibility of finding a universal medicine which should put
an end to disease and suffering and enable the adept to bring all
imperfect things to a state of perfection. The history of alchemy
is the history of a particular branch of the universal quest, the
quest of the unchanging.
In the later years of the eighteenth century, between 1770 and
1790, chemistry passed, at a bound, from being an empirical art to
becoming a science. The man who made the great transformation
was Antoine Laurent Lavoisier. With the work of the master we
are not concerned here.
From the time of Lavoisier to our own day, chemistry has
progressed, in the main, along four lines. For some years, chemists
concentrated their attention on one definite class of material
changes, the changes which happen when substances are burned
in the air. The knowledge which was gained of the changes of
composition and of properties during combustion incited and
guided chemists to a searching examination of the distinctive
properties of many different substances, and this examination
brought about the clarifying of the conception of definite kinds
of matter, and the application of this conception to the opening of
many paths of chemical enquiry. While these advances were being
made, a quiet member of the Society of Friends presented chemistry
with a marvellously delicate and penetrative instrument for further-
ing accurate knowledge of material changes. John Dalton made
## p. 272 (#302) ############################################
272
The Literature of Science [CH.
what seemed a small addition to the Greek atomic theory, an
addition which changed an interesting speculation into a scientific
theory. As the century went on, chemists began to elucidate
the connections between chemical events and physical phenomena.
The science of physical chemistry began.
Among those who investigated the phenomena of combustion
in the eighteenth, and early nineteenth, century, Priestley and
Cavendish are pre-eminent. Black was the first chemist to
make an accurate, quantitative examination of a particular,
limited, chemical change, and, by so doing, to give clearness to
the expression 'a homogeneous substance. ' The atomic theory
was Dalton's gift to science. From the many chemists who
amplified the work of Dalton, and used the conceptions of atom
and molecule to connect and explain new classes of chemical facts,
Williamson and Frankland may be selected as the representatives.
As workers in the borderland between chemistry and physics,
Graham and Faraday are specially to be remembered. The in-
vestigations of Davy touched and illuminated every side of
chemical progress.
Besides these men, who greatly enriched and advanced the
science of chemistry in the period under review, there were
many workers whose contributions cannot be considered here,
References are given in the bibliography to the writings of some
of them.
Joseph Priestley was a man of many gifts and a very versatile
mind. When a youth at an academy, he tells us that he 'saw
reason to embrace what is generally called the heterodox side of
almost every question. When about twenty-eight years of age,
he taught, in a school at Warrington, languages (he had a great
natural gift of tongues), oratory and criticism, elocution, logic,
natural phenomena, civil law and anatomy.
In the seventies of the eighteenth century, Priestley turned his
attention to different kinds of airs. He obtained and partially
examined many gases, but rarely troubled about separating them
completely from impurities. In August 1774, Priestley obtained a
large lens with which he concentrated the sun's rays on whatever
substance happened to come to his hand, with the object of finding
what air could be extracted from it. When he thus heated mer-
curius calcinatus per se (now called oxide of mercury), he obtained
an air in which a candle burned with a remarkably vigorous
flame. ' This result, he says, 'surprised me more than I can well
express. ' The new air was subjected to many tests; it always
6
## p. 273 (#303) ############################################
VIII] Priestley's Work on Gases
273
behaved in a very unexpected manner. He placed a mouse in his
new air; the mouse remained lively, and the air did not become
noxious. ' The results of other experiments caused Priestley
to lie awake through the night ‘in utter astonishment. ' At last,
he concluded that the new air was 'between four and five times as
good as common air. He regarded the new air as a very superior
kind of common air.
Priestley thought alchemically, not as a chemist. To the
alchemist, the properties of things were external wrappings which
might be removed from one thing and put round another, without
affecting the essential substance of either thing, which substance
it was the business of properties to hide from the uninitiated.
Priestley thought of different airs as identical, or nearly identical,
in substance, and only apparently different because of superficial
differences in the mantles, the properties, by which the essential
substance was concealed. When he obtained the air from burnt
mercury, he thought he had removed from common air something
which made it ‘noxious, vitiated, depraved, corrupt. ' He had not
learnt, what Black’s experiments, made twenty years before 1774,
might have taught him, that each particular, material thing is
known only by its properties. Priestley's forced explanation of the
facts which he himself discovered helped to convince investigators
that the notion of identity of substance hidden under differences
of properties is a great hindrance to the acquirement of accurate
knowledge of natural events.
Priestley could not get over his astonishment at the behaviour
of the new air. In science, one does well to be astonished; but,
to astonishment one must add investigation, to investigation,
reasoning, and, to reasoning, more investigation. Stopping at
astonishment, Priestley made his facts square with the theory that
dominated him, the theory of phlogiston. The phlogisteans taught
that something, which they had named phlogiston, the principle of
fire, rushes out of a burning substance as it burns. Phlogiston
was never captured. Priestley held that the elusive phlogiston is
a great corrupter of your airs or gases. He supposed that he had
deprived common air of this depraving principle; he named his
new gas dephlogisticated air. He invented many very ingenious
hypotheses to account for facts observed by himself. Had he
made a few accurate quantitative experiments, he might have
broken the toils of his favourite theory.
The French chemist Lavoisier saw the importance of Priestley's
discovery of dephlogisticated air, and, by a series of rigidly
18
E. L. XIV.
CH. VIII.
## p. 274 (#304) ############################################
274 The Literature of Science [ch.
>
quantitative experiments with tin and mercury, proved that, when
a substance burns in air, it combines with a constituent of the air,
which air-constituent is the gas prepared by Priestley. Lavoisier
called this gas oxygen, because many of its compounds are
acids.
Priestley's insatiable curiosity, his mental alertness, his im.
patience of details, were required for the advancement of chemistry,
no less than the passionless determination and the scrupulous
accuracy of Cavendish.
Henry Cavendish, of Peterhouse, was bred in the theory of
phlogiston, as Priestley was, and remained faithful to that theory,
as Priestley did. He thought of many airs, or gases, as more or less
phlogisticated forms of a few particular substances. Cavendish
described the explosion of a mixture of common air and inflammable
air (obtained by the action of acids on zinc) as one of the ways of
phlogisticating air. This process is accompanied by a decrease in
the volume of the interacting gases. Cavendish tried to discover
the cause of this decrease. He exploded accurately measured
volumes of dephlogisticated air (oxygen) and inflammable air
(hydrogen), and found that water was the sole product of the
change when dephlogisticated air was mixed with twice its volume
of inflammable air. The explanation which Cavendish gave of
this fundamentally important fact was confused and vague, because
he insisted on making the facts uphold the phlogistic theory.
Without knowing exactly what he had done, Cavendish had
determined the quantitative volumetric composition of water.
When the phlogistic theory had been swept away, the very
great importance of the accurate work of Cavendish became
manifest.
Joseph Black graduated as doctor of medicine in the university
of Edinburgh in 1755, presenting a thesis entitled Magnesia
alba, Quicklime, and other alcaline substances. That thesis is
probably the earliest example of a genuinely scientific chemical
investigation. Black proved that mild magnesia (now called
magnesium carbonate) loses weight when it is calcined; he deter-
mined the loss of weight; he proved that the solid substance
which remains after calcination has properties of its own which
distinguish it from mild magnesia; he showed that, during calci-
nation, an air, or gas, is given off, different from any air, or gas,
then known ; he examined, accurately, the properties of this gas,
which he called fixed air; and he reproduced the original quantity
of mild magnesia by dissolving the calcined magnesia in acid, and
>
## p. 275 (#305) ############################################
VIII) Black and Cavendish
275
adding fixed alkali (now called potassium carbonate), a substance
which he proved to give off fixed air when it is calcined. By his
experiments, Black proved mild magnesia to be composed of fixed
air united with calcined magnesia, and showed that each of these
three substances is a particular and definite kind of matter, dis-
tinguished from all other kinds of matter by constant qualities.
He also proved that the change which happens when chalk is
burned is exactly similar to the calcining of mild magnesia ; fixed
air is driven out of the chalk, and burnt lime—a perfectly
definite homogeneous substance-remains.
The work of Black prepared the way for the penetrative, ex-
perimental analysis of the phenomena of combustion ; it taught
chemists to use accurately observed properties of bodies as the
only means of distinguishing one body from another; it showed
that, if chemical investigation is to produce results of permanent
value, it must be quantitative; incidentally, by isolating and
examining fixed air, it began a new branch of chemistry, the study
of the changes of composition and properties which happen when
homogeneous gases interact.
Black and Cavendish were painstaking, methodical, un-
emotional, eminently clear-headed. Priestley was flighty, fitting
from one thing to another in his laboratory, always curious, never
working out his discoveries, unable to think chemically outside of
the theory which dominated him. Black, Cavendish and Priestley
greatly advanced the science of chemistry.
So long as chemists formed vague generalisations founded on
introspective speculations, they made little progress. It was by
concentrating their attention on a few limited occurrences, and
accurately examining these by quantitative experiments, that
chemists gradually gained clear conceptions which could be
directly used in the investigation of more complicated chemical
changes. “True genius,' Coleridge said, 'begins by generalising
and condensing; it ends in realising and expanding. ' The vague
generalising of the alchemists was followed by the condensing
work of Black and Cavendish, and by the suggestive discoveries of
Priestley. The time was approaching for realising and expanding.
In 1808, a small book appeared, entitled A new system of
Chemical Philosophy, Part I, by John Dalton. The influence of
that book on the development of chemistry, and of physics also,
has been very great.
Dalton delivered a lecture in Manchester, in 1803, wherein he
said 'An enquiry into the relative weights of the ultimate particles
18-2
## p. 276 (#306) ############################################
276
The Literature of Science [CH.
of bodies is a subject, so far as I know, entirely new; I have lately
been prosecuting this enquiry with remarkable success. ' Many of
Dalton's predecessors, both chemists and physicists, had used, in a
vague and general manner, the Greek conception of the atomic
structure of matter. Dalton showed how the relative weights of
atoms can be determined. By doing that, he brought down the
atomic theory to the solid earth, and made it a bold, suggestive,
stimulating guide ready for the use of chemists and physicists.
Dalton was not a great experimenter; he generally used the
results of other chemists' experiments. He was a scientific thinker,
characterised by boldness and caution. Dalton assumed, as
Lucretius had done long before him, that matter has a grained
structure ; that all the ultimate particles of each particular
homogeneous substance are identical, and differ in properties,
one of which is their weight, from the particles of all other
definite substances; he also assumed that the mechanism of
chemical changes, that is, changes wherein homogeneous substances
are produced different from those present when the changes began,
is the coalescence of atoms of different kinds to form new sorts of
atoms.
In order to find the relative weights of atoms, Dalton argued
as follows: Analyses and syntheses of water show that eight
grains of oxygen unite with one grain of hydrogen to form water.
If this change is the union of atoms of oxygen with atoms of
hydrogen, to form atoms of water, and if all the atoms of each
one of these three homogeneous substances are identical in weight
and other properties, it follows that an atom of oxygen is eight
times heavier than an atom of hydrogen. If we take the atomic
weight of hydrogen as unity—because hydrogen is lighter than
any other known substance—then the atomic weight of oxygen is
eight, and the atomic weight of water is nine.
In arriving at the conclusion that the atomic weight of oxygen
is eight, if the atomic weight of hydrogen is one, Dalton made the
assumption that a single atom of oxygen unites with one atom of
hydrogen to form an atom of water. He made this assumption
because it was simpler than any other. Had he chosen to suppose
that two atoms of hydrogen unite with one atom of oxygen, he
must have assigned to oxygen the atomic weight sixteen, and to
water the atomic weight eighteen.
To make Dalton's method perfectly general, and quite conclusive
in its results, it was necessary to find means for fixing the relative
weights of the atoms formed by the union of other, simpler, atoms;
## p. 277 (#307) ############################################
VII] The Atomic Theory. Dalton and Others 277
it was also necessary to find means of determining the number of
atoms of each kind which unite to form a more complex atom.
A general method for solving these two problems was given to
chemistry in 1811–12 by an Italian physical chemist named
Avogadro, who brought into science the notion of a second order
of minute particles, supplementing the conception of atom by that
of molecule.
It is not possible in this brief sketch to indicate the many new
fields of investigation which were opened, and made fruitful, by
the Daltonian atomic theory. From the many workers who used
this theory as a means for pressing forward along new lines of
enquiry, two may be selected, since their work is typical of much
that was done in chemistry during the first half of the nineteenth
century.
Alexander Williamson strove to make chemists realise the need
of using the Avogadrean molecule as well as the Daltonian atom.
By his work on etherification, and by other experimental investiga-
tions, as well as by reasoning on his own results and those obtained
by other chemists, Williamson demonstrated the fruitfulness of
the notion of the molecule. He endeavoured to determine the
relative weights of molecules by purely chemical methods. These
methods proved to be less satisfactory, and much less general,
than the physical method which had been described by Avogadro.
The conception of equivalency, that is, equal value in exchange,
of determinate weights of different homogeneous substances, has
been very helpful in chemistry.
In 1852, Edward Frankland
applied the notion of equivalency to the atoms of elements, that
is, homogeneous substances which have not been separated into
unlike parts. He arranged the elements in groups, the atoms of
those in any one group being of equal value in exchange, inasmuch
as each of these atoms combines with the same number of other
atoms to form molecules.
When Frankland's conception had been developed, and the
method of determining the equivalency of atoms made more
definite and more workable, a vast new field of enquiry was
opened, a eld which has proved remarkably fruitful both in
purely scientific work, and in applied chemistry. It is not an
exaggeration to say that the great industry of making aniline
colours is an outcome of the notion of atomic equivalency intro-
duced by Frankland into chemical science.
The words element and principle were used by the alchemist
as nearly synonymous; both words were used vaguely. The
## p. 278 (#308) ############################################
278
[ch.
The Literature of Science
meaning given to the term element, by Lavoisier, towards the
end of the eighteenth century—a definite kind of matter which
has not been decomposed, that is, separated into unlike parts-
was elucidated, and confirmed as the only fruitful connotation
of the term by the work of Sir Humphry Davy on potash and soda
in 1808.
Humphry Davy was the most brilliant of English chemists.
He was the friend of Wordsworth and Sir Walter Scott. Lockhart
says that the conversation of Davy and Scott was fascinating and
invigorating. Each drew out the powers of the other.
6
I remember William Laidlaw whispering to me, one night when their
‘rapt talk 'had kept the circle round the fire until long after the usual bedtime
of Abbotsford-Gude preserve us! this is a very superior occasion 1! '
Davy sent an electric current through pieces of potash and soda;
the solids melted, and 'small globules, having a high metallic
lustre, and being precisely similar in visible characters to quick-
silver, appeared. By burning the metal-like globules, Davy
'
obtained potash and soda. Making his experiments quantitative,
weighing the potash and the soda before passing the current, and
the potash and soda obtained by burning the metal-like products
of the first change, he proved that potash and soda, which, at that
time, were classed with the elements, are composed each of a metal
combined with oxygen. The new metals—potassium and sodium-
are soft and very light, and instantly combine with oxygen when
they are exposed to the air.
Everyone had been accustomed to think of a metal as a heavy,
hard solid, unchanged, or very slowly changed, by exposure to air.
Had chemists strictly defined the term metal, they could not have
allowed the bases of potash and soda (as Davy called the new sub-
stances) to be included among metals. Happily, the definitions of
natural science are not as the definitions of the logician; they are
descriptive summaries of what is known, and suggestive guides to
further enquiry.
As every attempt to separate potassium and sodium into unlike
parts failed, Davy put them into the class elements ; he said—Till
a body is decomposed, it should be considered as simple. '
In 1810, Davy investigated a substance concerning the
composition of which a fierce controversy raged. Oxymuriatic
acid was said by almost all chemists at that time to be a compound
of oxygen with an unknown base. No one had been able to get
1 Life of Sir Walter Scott (6 vols. , 1900), vol. III, p. 403.
## p. 279 (#309) ############################################
a
6
VII] Davy. Physical Chemistry
279
oxygen from it, or to isolate the base supposed to be a constituent
of it. By putting away, for the time, all hypotheses and specula-
tions, and by conducting his experiments quantitatively, Davy
showed that oxymuriatic acid is not an acid, but is a simple
substance, that is, a substance which is not decomposed in any
of the changes it undergoes. He proposed to name this simple
substance chlorine ; a name, Davy said, 'founded upon one of its
obvious and characteristic properties-its colour. ' Davy re-
marked— Names should express things not opinions. '
Davy thought much about the connections between chemical
affinity and electrical energy, and investigated these connections
by well planned experiments. In 1807, he said—May not the
electrical energy be identical with chemical affinity ? ' He used
the expressions—'different electrical states,' and 'degrees of
exaltation of the electrical states,' of the particles of bodies.
Recent researches into the subject of chemical affinity have
established the great importance of the conceptions adumbrated
by Davy in these expressions.
Chemistry, the study of the changes of composition and
properties which happen when homogeneous substances interact,
has always been closely connected with physics, the study of the
behaviour of substances apart from those interactions of them
in which composition is changed. Among the earlier physical
chemists, Graham occupies an important place.
Thomas Graham was a shy, retiring man, most of whose life
was spent in his laboratory. There is a tradition in the Glasgow
institution, where he taught chemistry, in his younger days, before
moving to London (in his later years he was master of the mint),
that, when he came into the lecture theatre, to deliver his first
lecture to a large audience, he looked around in dismay and fled.
Graham established the fundamental phenomena of the diffusion
of gases and of liquids; he distinguished, and applied the distinction,
between crystalloids, solutions of which pass through animal and
vegetable membranes, and colloids, which do not pass through
those membranes. The investigation of the behaviour of colloidal
substances has led, in recent years, to great advances in the
knowledge of phenomena common to chemistry, physics and
biology.
Electrochemistry, the study of the connections between chemical
and electrical actions, has been productive, in recent years, of more
far-reaching results than have been obtained in any other branch
of physical chemistry. Much of what has been done in the last
a
## p. 280 (#310) ############################################
280 The Literature of Science [CH.
half-century is based on the work of Faraday, and, indirectly, on the
suggestion of Davy. Both were men of genius, that is, men who
see the central position of the problem they are investigating, who
seize and hold that position until the problem is solved, letting the
surface phenomena, for the time, go to the dogs, what matters? '
Men of genius work from the centre outwards.
To Michael Faraday, we owe the fundamental terms of electro-
chemistry. The separation of a salt into two parts by the electric
current, he called electrolysis ; the surfaces from which the current
passes into, and out of, an electrolysable compound, he named
electrodes; the substances liberated at the electrodes, he called
ions. Faraday measured the chemical power of a current' by
the quantities of the ions set free during a determinate period
of electrolysis. Taking as his unit the quantity of electricity
which liberates one gram of hydrogen from an electrolysable
compound of that element, he showed that the weights of different
ions liberated from compounds by unit quantity of electricity are
in the proportion of their chemical equivalents. Using the language
of the atomic theory, Faraday declared that 'the atoms of bodies
which are equivalent to each other in their ordinary chemical action
have equal quantities of electricity mutually associated with them. '
In 1834, Faraday said— The forces called electricity and
chemical affinity are one and the same. ' Faraday distinguished
the intensity of electricity from the quantity of it, and indicated
the meaning of each of these factors. One would not greatly
exaggerate if one said that the notable advances made in the
last quarter of a century in the elucidation of chemical affinity
are but developments and applications of Faraday's pregnant
work on the two factors of electrical energy.
The results established by Faraday have led to the conception
of atoms of electricity, a conception which has been of great
service in advancing the study of radioactivity. Faraday's results
have also been the incentives and guides in researches which go
to the root of many problems of the physical sciences, and of not
a few of the biological sciences also.
At the time of the foundation of the Royal Society, chemistry
was a conglomeration of more or less useful recipes, and a dream
of the elixir. Today, chemistry is becoming an almost universal
science. Happily, chemists still dream.
## p. 281 (#311) ############################################
VIII]
Biology
281
C. BIOLOGY
6
Although science, during the eighteenth century, was, like
many other intellectual activities in our country, more or less
in abeyance, an attempt has been made, in the following pages,
to carry on the subject in the present chapter from that which
appeared in a previous volume (VIII) of this History.
'The Royal Society of London for Improving Natural Know-
ledge, one of the oldest scientific societies in the world and
certainly the oldest in the empire, was formally founded in 1660,
and received its royal charter of incorporation two years later.
At a preliminary meeting, a list had been prepared of some forty
names of such persons as were known to those present whom
they judged willing and fit to joyne. . . in the designe,' and among
these names we find those of 'Mr Robert Boyle, Sir Kenelme
Digby, Mr Evelyn, Dr Ward, Dr Wallis, Dr Glisson, Dr Ent,
Dr Cowley, Dr Willis, Dr Wren' names whose owners have been
dwelt upon in volume VIII.
Thus, for the first time in our country, the study of science was,
to a degree, organised and its advancement promoted, not only
by periodical meetings where experiments were conducted and
criticism freely offered, but by the collection of scientific books,
which still remain at Burlington house, and of 'natural objects,
which have for long formed part of the British Museum's
collections.
So Virtuous and so Noble a Design,
So Human for its Use, for Knowledge so Divine,
as Abraham Cowley, the laureate of the new movement, wrote,
was, in part, a protest against the credulity and superstitions of
a credulous and superstitious age, and the word ‘natural, as
used in the charter, was used in deliberate opposition to “super-
natural,' the aim of the society being, at any rate in part, to
discourage divination and witchcraft.
We have said something about the brilliant band of physio-
logists, headed by Harvey, who made the Stewart period remarkable
in the annals of English science; though there were then other
biologists less gifted than Harvey, but still leaders in their several
fields. The recent invention of the microscope had given a great
impetus to the study of the anatomical structure of plants and,
later, of animals; and, in relation to this, we must not overlook
## p. 282 (#312) ############################################
282 The Literature of Science [CH.
the work of Nehemiah Grew, who, with the Italian Malpighi, may
be considered a co-founder of the science of plant-anatomy.
Nehemiah Grew studied at Pembroke hall, Cambridge, and after-
wards took his doctor's degree at Leyden. He published numerous
treatises dealing with the anatomy of vegetables, and with the
comparative anatomy of trunks, roots, and so forth, illustrated
by admirable, if somewhat diagrammatic, plates. Although
essentially an anatomist, he made certain investigations into
plant physiology and suggested many more. Perhaps his most
interesting contribution to botany, however, was his discovery
that flowering plants, like animals, have male and female sexes.
It seems odd to reflect that this discovery is only about 250 years
old. When Grew began to work, the study of botany was in a
very neglected condition—the old herbal had ceased to interest,
and, with its contemporary, the bestiary, was disappearing from
current use, while the work of some of Grew's contemporaries,
notably Robert Morison and John Ray, hastened their dis-
appearance. Of these two systematists, Ray, on the whole, was the
more successful. His classification of plants obtained in England
until the latter half of the eighteenth century, when it was gradually
replaced by the Linnaean method of classification.
But Ray has other claims on our regard. He and Francis
Willughby, both of Trinity college, attacked a similar problem in
the animal kingdom. Willughby was the only son of wealthy and
titled parents, while Ray was the son of a village blacksmith.
But the older universities are great levellers, and Ray succeeded
in infusing into his fellow student at Cambridge his own genuine
love for natural history. With Willughby, he started forth on his
methodical investigations of animals and plants in all the accessible
parts of the world. Willughby died young and bequeathed a
small benefaction and his manuscripts to his older friend. After
his death, Ray undertook to revise and complete his Ornithology,
and therein paid great attention to the internal anatomy, to the
habits and to the eggs of most of the birds he described. He, further,
edited Willughby's History of Fishes, but perpetuated the mistake
of his predecessors in retaining whales among that group.
In
rather rationalistic mood, he argues that the fish which swallowed
Jonah must have been a shark. Perhaps the weakest of their
three great histories—the History of Insects—was such owing
to the fact that Ray edited it in his old age.
Ray was always a fine field naturalist, and his catalogues of
Cambridgeshire plants long remained a classic. We may, perhaps,
## p. 283 (#313) ############################################
VIII] Ray, Willughby and Hooke 283
sum up the contributions of this great naturalist in the words of
Miall :
During his long and strenuous life he introduced many lasting improve-
ments-fuller descriptions, better definitions, better associations, better
sequences. He strove to rest his distinctions upon knowledge of structure,
which he personally investigated at every opportunity. . . . His greatest
single improvement was the division of the herbs into Monocotyledons and
Dicotyledons 1
Robert Hooke, a Westminster boy and, later, a student at
Christ Church, was at once instructor and assistant to Boyle.
The year that the Royal Society received their charter, they
appointed Hooke curator, and his duty was 'to furnish the
Society' every day they met with three or four considerable
experiments. This amazing task he fulfilled in spite of the fact
that the fabrication of instruments for experiments was not
commonly known to workmen,' and that he never received above
£50 a year and that not certain. ' Hooke was a man of amazing
versatility, very self-confident, attacking problems in all branches
of science, greatly aiding their advance, but avid of fame.
In person but dispicable, being crooked and low in nature and as he grew
older more and more deformed. He was always very pale and lean and
latterly nothing but skin and bone 2.
His active, jealous mind conceived that almost every discovery of
his time had been there initiated; and this anxiety to claim ‘priority'
induced Newton to suppress his treatise Optics until after the date
of Hooke's death. His book Micrographia, 'a most excellent
piece, of which I am very proud,' as Pepys has it, is the record of
what a modern schoolboy newly introduced to the microscope would
write down. Yet he was undoubtedly, although not a lovable
character, the best 'mechanic of his age. '
British physiology, which had started magnificently with
Harvey, and had continued under Mayow, de Mayerne and others,
was carried forward by Stephen Hales, at one time fellow of
Corpus Christi college, Cambridge, and for years perpetual curate
at Teddington. He was a born experimenter, and, as a student,
worked in the 'elaboratory of Trinity College, which had been
established under the rule of Bentley, ever anxious to make his
college the leader in every kind of learning. Sachs has pointed
out that, during the eighteenth century, the study of the anatomy
of plants made but little progress ; but there was a very real
1 The Early Naturalists, L. C. Miall, London, 1912.
? Waller's Life of Hooke, 1705.
## p. 284 (#314) ############################################
284 The Literature of Science [CH.
advance in our knowledge of plant physiology. This, in the main,
was due to Hales; he investigated the rate of transpiration and
held views as to the force causing the ascent of sap which have
recently come to their own; he recognised that the air might
be a source of food for the plant and connected the assimilative
function of leaves with the action of light,' though he failed to
find the mode of the interaction. He worked much on gases, and
paved the way for Priestley and others by devising methods of
collecting them over water. Hales, this 'poor, good, primitive
creature,' as Horace Walpole called him, was not less remarkable
as an investigator of animal physiology, and was the first to
measure the blood-pressure, and the rate of flow in the capillaries.
Sir Francis Darwin states:
In first opening the way to a correct appreciation of blood-pressure Hales'
work may rank second in importance to Harvey's in founding the modern
science of physiology.
He was, further, a man of 'many inventions,' especially in the fields
of ventilation and hygiene.
The beginning of our period coincides with the formation of
public museums. Previous to the Stewart times, collections of
‘natural objects' were, for the most part, housed in churches, in the
houses of the great, in coffee-houses and in the shops of apothe-
caries; but now public libraries were being established, and, in many
of these, botanical, geological and especially zoological specimens
found a home. In more than one Cambridge college, the library
still gives shelter to a skeleton, a relic of the time when anatomy
was taught within the college walls; and, at this day, the curious,
and, at times, inconvenient, yoke joining the museum at South
Kensington with the museum in Bloomsbury testifies to this
primitive state of affairs.
In 1728, John Woodward bequeathed his 'Fossils, a vast quan-
tities of Ores, Minerals and Shells, with other curiosities well
worth viewing' to Cambridge university; it was housed in the
university library and formed the nucleus about which the present
magnificent museum has collected. For many years, the Royal
Society maintained a museum which, at one time, contained 'the
stones taken out of Lord Belcarre's heart in a silver box,'. . .
‘a petrified fish, the skin of an antelope which died in St James'
Park, a petrified foetus' and 'a bottle full of stag's tears. ' The
trustees of Gresham college assigned the long gallery as a home
for these and other 'rarities'; but, when the society, in 1781,
migrated to Somerset house, the entire collection was handed over
## p. 285 (#315) ############################################
VIII] Origin of Museums
285
to the British Museum. The charter of the last named is dated
1753, and its beginnings were the library of Sir Robert Cotton,
wbich the nation had purchased in 1700, and the collections of
Sir Hans Sloane, which were now purchased with the proceeds of
a lottery, set on foot for this purpose. The collections of this
General Repository,' as the act of 1753 called the museum, were
kept together until the middle of the nineteenth century, when,
after long delay, the natural history objects were transferred to
South Kensington and housed in a building which, in all respects,
was worthy of the Board of Works of the time.
John Tradescant and his son of the same name accumulated
and stored in south Lambeth a 'museum which was considered to
be the most extensive in Europe at that time. It was acquired in
1659 by Elias Ashmole, and, with his own collections, passed
by gift, twenty-three years later, to Oxford university, the whole
forming the nucleus of the present Ashmolean museum.
Want of space precludes the consideration of other museums;
but it may be remarked that the earlier collectors got together
their treasures much as schoolboys now collect, their taste was
universal and no rarity was too trivial for their notice. Such collec-
tions excited popular interest, and 'a museum of curiosities' was
often an added attraction to the London coffee-house. At the end of
the eighteenth century, the coffee-house part of the enterprise was
dropped, and the museum, with an entrance-fee and a priced cata-
logue, formed a source of revenue to many a collector, most
of whom were not too scrupulous in their identifications. The
dime museums in the Bowery, New York, are their modern
successors. These museums were of little scientific or educational
value ; at best, they stimulated the imagination of the uninformed,
or allowed a child to see with his own eyes something he had read
about in his books. The normal, as a rule, was passed by, the
abnormal treasured. Ethnographical objects were collected, not
so much to arouse in the spectator a desire to study seriously
‘y® beastlie devices of yo heathen' as to excite and startle him
with their rough unfinish, on the one hand, and their high finish
on the other. The collections of the museums were ill arranged,
inaccurately labelled and inaccessible to students; the staff
were wholly inadequate and mainly dependent for their living
on admission fees. It was not until the nineteenth century
was well advanced that a systematic and scientific attempt
was made to identify specimens accurately, to arrange them
logically, to label them fully and, further, to collect in the
6
## p. 286 (#316) ############################################
286 The Literature of Science [CH.
background, unseen by the fleeting visitor, vast accumulations
of material for the investigation of the genuine student and
researcher.
Museums as centres of real education, not as places of wonder
and vacant amazement, are almost affairs of our time, and it
was not until the twentieth century that official guides were
appointed to explain their treasures to the enquiring visitor.
Even today, the system of weekly lectures on the contents of a
museum which obtains largely on the other side of the Atlantic
is, with us, only beginning.
We must not omit to mention the magnificent museum of the
Royal college of Surgeons, in London, which incorporates the
Hunterian collection brought together by John Hunter, and which
has been growing ever since his time. Of its kind, it is without a
rival in the world.
During the seventeenth century, men of science still, to a
great extent, remained the gifted amateurs they were at the time
of the foundation of the Royal Society; and yet they were very
successful in establishing many institutions which had a greater
effect on the advance of biological sciences than their founders
foresaw.
Towards the beginning of the seventeenth century, the Oxford
botanic garden had been founded (1621), which was followed, in
1667, by the opening of the Edinburgh botanic garden, and, in 1673,
by the foundation of the Chelsea physic garden, by the Apothe-
caries' company.
At the beginning of the eighteenth century,
Glasgow followed suit. By this time, many of the universities had
chairs of botany, and botany and anatomy were the first biological
sciences represented by professorial chairs in this country. In
1724, a chair was established at Cambridge, with Bradley as
its first professor ; but he and his immediate followers had little
success and, for the most part, were non-resident. Oxford
followed, in 1734, and Dillenius was the first to occupy the chair,
which had been founded by William Sherrard. The botanic
garden at Oxford, however, had been in existence for many years.
At Cambridge, it was not till 1759 that Walker founded the
botanic garden, which, at that time, occupied the northern site
of the present museums of science. The fine specimen of the
Sophora tree, the tree which yields the Chinese imperial yellow
dye, is the last and only memorial of this old botanic garden.
In 1765, Kew gardens, originally in possession of the Capel family,
were combined with Richmond gardens, then occupied by the
## p. 287 (#317) ############################################
viii] Botanic Gardens and Learned Societies 287
princess Augusta, widow of Frederick, prince of Wales. In fact,
this lady may be regarded as the foundress of Kew, which, since
her time, has played the leading part in the dissemination of
botanical knowledge throughout the world.
In the latter half of the eighteenth century, the Linnaean
system of classification had been generally adopted in Great
Britain, and, in the year 1783, Sir James Edward Smith secured,
from the mother of Linnaeus, for £1050, the entire Linnaean
collections. These did not, however, reach these islands without
an effort on the part of the Swedish government to retrieve
them. Indeed, it sent a man-of-war after the ship which
transported them.
Following on this acquisition, Smith, in 1788, founded the
Linnaean society, the immediate effect of which, perhaps, was
to bring about a revolution in the mode of publishing scien-
tific literature From the first, the Linnaean society issued
journals and transactions instead of books or treatises; their
publications took the form of memoirs read before the society.
In this respect, the Linnaean society set a fashion which has been
consistently followed by the numerous societies which since have
sprung up.
The Royal Society had taken all science as its province, and
nothing in natural history was alien to the activities of the
Linnaean society ; but, with the beginning of the nineteenth
century, societies began to spring up in the metropolis which
devoted their energies to the advancement of one science alone.
The earliest effort was that of the Royal Horticultural society,
founded in 1803. Its first secretary was Joseph Sabine, to whom
much of its earlier success was due. For a time, it undertook
the training of gardeners and also sent collectors to foreign
countries in search of horticultural rarities. It still does much
for horticulture, especially by its very successful flower-shows.
The Geological society of London was founded in 1807. It was
partly the outcome of a previous club known as the Askesian
society, and among the more prominent founders were William
Babington, Humphry Davy, George Greenough and others. The
meetings were at first held at the Freemasons' tavern. The
society, like many other learned societies, now has rooms at
Burlington house.
The Zoological society of London for the advancement of
zoology and animal physiology, and for the introduction of new
and curious subjects of the animal kingdom was founded in
## p. 288 (#318) ############################################
288
[ch.
The Literature of Science
1826 by Sir Stamford Raffles, the wellknown traveller and governor
in the east and the godfather of Rafflesia, J. Sabine, N. A. Vigors
and other eminent naturalists. It was incorporated by royal
charter in 1829.
The Royal Botanic society was founded in 1839, and was
granted an area of eighteen acres within the inner circle of
Regent's park, and here Marnock laid out the gardens very much
as they still are. Shortly after its establishment, annual exhi-
bitions or flower-shows were begun, and such exhibitions, not
entirely confined to flowers, are still one of the features of the
society.
Another society which has played a most useful part in the
promotion of science is the Cambridge Philosophical society,
founded in the year 1819, the only society outside the capital
towns which possesses a royal charter. About the same time,
the Dublin society (founded in 1731) assumed the title royal
The Edinburgh Royal society was founded in 1783; the date of its
revised charter is 1811. Many other societies in our chief towns
did much to advance the cause of science; but they are too
numerous to record here.
Another institution which embraced all branches of science was
the British Association for the Advancement of Science, which was
due largely to the enterprise of Brewster, Babbage and Herschel
It held its first meeting in York in the year 1831. The objects of
its founders were
to give a stronger impulse and a more systematic direction to scientifie
enquiry, to promote the intercourse of those who cultivate science in different
parts of the British Empire with one another, and with foreign philosophers,
to obtain a more general attention the objects of science, and the removal
of any disadvantages of a public kind, which impede its progress.
With certain exceptions, the books on biology during the last
half of the eighteenth century and the beginning of the nineteenth,
were largely treatises on classification, or on the practical applica-
tion of the knowledge of plants, such as medical and agricultural
works. It was during this period, too, that certain magazines
were started. Curtis founded The Botanical Magazine in the
year 1787. But the great increase of scientific journals only
began some fifty years later; many of those dealing with different
branches of biological science were first published about the
middle of the nineteenth century. Among them may be men-
tioned the following, with the date of their first appearance:
The Annals and Magazine of Natural History, 1841 ; The
## p. 289 (#319) ############################################
VIII] Scientific Journals and Exploration 289
Zoologist, 1843 ; Quarterly Journal of Microscopical Science,
1853; The Journal of Horticulture, 1862; The Geological
Magazine, 1864; The Journal of Anatomy and Physiology,
1866.
on the motion of waves in a canal, and the deduction of the
geometrical laws of sound and light from the undulatory theory.
In these writings, he showed remarkable physical insight in the
applications of his analysis. His memoirs on the propagation of
light in a crystalline medium, published in 1839, rest on the
assumption that the ether in a crystal resembles an elastic solid
unequally pressed in different directions by unmoved ponderable
matter—a conception which, later, was to lead to remarkable
developments. Few writings have been more fruitful than those
of Green. They led MacCullagh and Cauchy to revise their theories
of optics, and they profoundly impressed Stokes and Kelvin, whose
work we now proceed to describe.
(Sir) George Gabriel Stokes spent his life at Cambridge, where
he held the Lucasian chair for over half a century. Through his
long tenure of the secretaryship of the Royal society, he acted as
the friend and guide of innumerable young authors, for, by virtue
of his office, he saw the manuscripts of all papers on mathematics
and physics, and freely placed at the disposal of the writers his
unrivalled knowledge of physics and mathematics : thus, a con-
siderable proportion of his work appears under the names of other
writers. He began his scientific career under the influence of
Green's writings. It is difficult to describe his researches in
general terms. The most important of them are concerned with
optics, hydrodynamics and geodesy. In optics, he was mainly
responsible for the explanation of fluorescence, and only just
missed being the first to propound the true explanation of
Fraunhofer's lines; he subjected diffraction to mathematical
analysis ; in hydrodynamics, we owe to him the modern theory
of viscous fluids, and he wrote on the properties and constitution
of the ether. His work in pure mathematics, especially on the
convergence of series, was also of importance. Stokes was an
excellent man of affairs-he sat for a time in the house of
commons—but his gift of silence prevented his exercising among
strangers the full influence which his abilities deserved. He was
the intimate friend of Kelvin and Maxwell, and to his deliberate
judgment on scientific matters Kelvin always yielded.
(Sir) William Thomson, later raised to the peerage under the
title baron Kelvin, was another graduate of Cambridge of this
## p. 267 (#297) ############################################
VIII]
Kelvin
267
period. (To avoid the confusion of the use of two names we will
here refer to him as Kelvin. ) In 1846, the year following on
his first degree, he accepted a professorial chair at Glasgow, but
he always kept in touch with his mathematical friends in Cambridge.
Probably, he exercised a wider influence in the world at large
than any of his scientific contemporaries; but his interests were so
catholic that it is not easy to give any connected account of them.
He possessed an almost intuitive power of realising fundamental
principles. Throughout his life, ideas seem to have come to him
so rapidly as to give him insufficient time for their effective
development; hence, the student will search in vain among his
papers for complete and systematic expositions of his discoveries.
He began his career under the influence of Fourier, Green and
Faraday. Electricity was his favourite subject of research. The
writer of this sketch has heard him illustrate the progress in this
subject by the fact that, in his early life, he was accustomed to
explain his conclusions in it by analogies drawn from the theory
of heat; but, before he died, problems in heat were commonly
illustrated by analogous questions in electricity. Kelvin wrote
at length on the subject of electromagnetic fields, put forward
numerous suggestions about the constitution of ether and matter,
and laid the foundation for a scientific system of measurement of
electrical quantities. · Hydrodynamics, elasticity and thermo-
dynamics were other subjects on which he wrote, and his papers
on energy and entropy were of far-reaching importance. We
cannot leave Kelvin's work without mentioning the appearance,
n 1867, of the treatise on natural philosophy by himself and his
friend Peter Guthrie Tait, of Cambridge and Edinburgh. In spite
of Tait's collaboration, this book presents an unfinished aspect;
but it is suggestive, and it widely affected modes of physical
thought throughout Europe.
The theory of signalling by cable originated in a correspondence
between Kelvin and Stokes in 1854, and was elaborated by Kelvin
and Kirchhoff, while to the former are largely due the practical
applications of it. The earliest successful submarine cable laid
between England and the continent dates only from 1851. Owing
to the large capacity of the cable and the soakage into the
insulating material, powerful currents had to be used before
sensible effects could be obtained, and these difficulties increased
with the length of the cable. Accordingly, when, in 1857, a cable
was laid to America, the operators deemed it necessary to use
electricity of a high tension, with the result that the insulation
## p. 268 (#298) ############################################
268 The Literature of Science [Ch.
a
was ruptured. Subsequently, Kelvin was given a free hand in
arranging a system for use with a later cable. He evaded the old
difficulties by developing extreme sensitiveness in the receiving
instruments and applied the methods of Gauss and Weber for
indicating the minute motions of the oscillating needle by the
reflection of a ray of light from it, in effect employing a long
non-material pointer. In 1870, he substituted for this method
a syphon-recorder which printed the message ; and this instrument
is still sometimes used. He held that the transmission of signals
along the wire of a submarine cable was due to an actual dis-
turbance in the wire : whereas, according to the modern theory,
propounded by Maxwell, the function of the wire is merely to
guide the disturbance resident in the surrounding dielectric.
Kelvin was a keen yachtsman, and was thus led to take up the
problem of compasses ; he also bore an active part in the develop-
ment of electrical engineering. He was the owner of several
patents connected with these practical applications of science.
We have next to mention one whose work has had so important
an influence on the subsequent growth of the subject as to make
it the beginning of a new epoch. This was James Clerk Maxwell
-the most modest of men-another member of the Cambridge
school, who, for the last eight years of his life, occupied
in the university the then newly created chair of experimental
physics.
Since the time of Descartes, natural philosophers had never
ceased to speculate on the processes by which gravity, light and
electricity are transmitted through space. So far as electricity
is concerned, the idea of lines of force in a continuous medium is
due to Faraday. Kelvin, as a young man, had suggested that
electric force might be transmitted through a medium, somewhat
as elastic displacements are transmitted through an elastic solid.
This idea was taken up by Maxwell, who, in 1856, elaborated the
analogies offered by the flow of a liquid, and, five years later,
devised a mechanical model of electromagnetic action. He now
brought forward a series of arguments to show that an electric
current was a phenomenon of translation, magnetism one of
rotation and the electrostatic state one of strain of the ether.
These conclusions led him to assert that light consists of trans-
verse waves of the same medium as that required for the
explanation of electric and magnetic phenomena. On this theory,
all currents are closed; magnetic energy is the kinetic energy
of the ether, and electric energy the energy of 'strain of the ether.
## p. 269 (#299) ############################################
VIII)
Maxwell's Electromagnetic Theory 269
These views were presented, as a whole, in 1864. Further ex-
tensions and developments of the theory followed, and the whole
was set out in his treatise published in 1873. This celebrated
work is far from easy to read, and the exposition is not
systematic, but it may be said that the fundamental ideas are now
universally accepted, and most of the work of his successors has
been built on the foundation here laid. The theory was based on
Faraday's ideas; but it required a trained mathematician to give
the final form to his conceptions and to deduce their consequences.
Hence, the theory is properly associated with Maxwell's name.
Maxwell, also, took a considerable part in framing a standard
system of electrical measurements. He contributed largely to the
kinetic theory of gases, and, incidentally, to theories on the con-
stitution of matter.
A large part of the history of mathematical physics during the
last quarter of the nineteenth century consists of the completion
and extension of Maxwell's electromagnetic theory. No incon-
siderable part of this is due to his successors at Cambridge, and to
describe recent researches in physics without mentioning the names
of lord Rayleigh, Sir Joseph John Thomson and Sir Joseph Larmor
is almost impossible; here, however, we must content ourselves
with a very brief account of the general line of investigation
followed in the last part of the period covered by the present
section.
It has already been pointed out that Maxwell's exposition of
his electromagnetic theory of light was neither systematic nor
complete. A curious omission in it was the absence of any
explanation of reflection and refraction ; this was supplied by
Helmholtz. The problem of the effects produced by the translation
of electric charges, raised by the same investigator, was solved by
the researches of the present Cavendish professor at Cambridge,
George Francis FitzGerald of Dublin, and others : in the mathe-
matical development of the theory, which now proceeded apace,
they, again, took a prominent part. In 1883, FitzGerald explained
a system of magnetic oscillators by which radiant energy could
be obtained from electrical sources, thus confirming Maxwell's
theoretical conclusion that light was an electromagnetic phe-
Some of Maxwell's assumptions on which he had
based his theory still remained unconfirmed; but, a year or two
later, the theory was placed on a firmer experimental basis by
Hertz. The results, incidentally, led to the introduction of wire-
less telegraphy.
nomenon.
## p. 270 (#300) ############################################
270 The Literature of Science [CH.
The question of the conduction of electric discharges through
liquids and gases had been raised by Faraday. It was now taken
up seriously, and various types of rays, cathode rays, Röntgen
rays, etc. , were discovered. These researches led to new views on
the constitution of matter. The investigations began with a
theory of electrons, and, finally, led to the view that every so-
called atom is formed by a combination of two elements in varying
proportions, and that, possibly, these two elements are to be
identified with forms of electricity-one of the most far-reaching
hypotheses propounded in recent times.
The efforts to extend the theory of the electromagnetic field to
cases where heavy masses are in motion introduces the difficult
question as to whether the ether round and in bodies is affected
by their motion, and to this theory of relativity much attention
is now being paid.
One of the striking features of the Victorian period has been
the equipment of large laboratories where experiments can be
carried out by students with an accuracy wholly impossible in
former days. Two of the earliest of these were built at Oxford
and Cambridge, the former known as the Clarendon laboratory
in 1872, the latter, known as the Cavendish laboratory, being
the gift of the seventh duke of Devonshire. In the latter,
Clerk Maxwell taught and has been succeeded by professors
not less distinguished. The existence of such laboratories in
seats of learning has profoundly affected the teaching of the
subject by training large numbers of competent observers, besides
calling forth in ever widening circles an intelligent interest in
physical studies.
It is not, we think, too much to say that the work in physics of
the Victorian period has completely revolutionised the subject,
and, both on its theoretical and practical sides, far exceeds in value
that previously done in any period of similar extent. The theory
of gravitation was the great achievement of the Newtonian school.
In the following century, physical optics and, later, the nature of
ether attracted most attention from philosophers, while practical
men developed the steam-engine and studied the theory of heat.
The Victorian age has seen electricity raised to the rank of an all-
embracing science, and applied to innumerable industrial uses,
power-engines, lighting, heating, telegraphy, telephones. Other
important scientific and industrial applications relate to photo-
graphy and spectrum analysis ; the development of the turbine-
engine; the invention of the internal combustion-engine, with its
## p. 271 (#301) ############################################
VIII]
Chemistry
271
numerous uses in transport on land and water; the introduction
of submarine boats, and heavier-than-air flying-machines ; and
the use of wireless telegraphy. In this chapter, however, bare
reference to these practical applications must suffice.
B. CHEMISTRY
Chemistry has always busied itself with the changes of
material things. By working in metals and precious stones, by
making colours, by producing things used by artists to give
delight to themselves and others, by fashioning natural materials
into things useful to men, by concocting potions which had strange
effects on the bodies and minds of those who swallowed them, by
doing these things and things like these, chemists slowly amassed
much knowledge, knowledge, however, which was fragmentary and
disconnected. The strange changes which chemists discovered
impelled the more ardent and adventurous among them to dream
of the possibility of finding a universal medicine which should put
an end to disease and suffering and enable the adept to bring all
imperfect things to a state of perfection. The history of alchemy
is the history of a particular branch of the universal quest, the
quest of the unchanging.
In the later years of the eighteenth century, between 1770 and
1790, chemistry passed, at a bound, from being an empirical art to
becoming a science. The man who made the great transformation
was Antoine Laurent Lavoisier. With the work of the master we
are not concerned here.
From the time of Lavoisier to our own day, chemistry has
progressed, in the main, along four lines. For some years, chemists
concentrated their attention on one definite class of material
changes, the changes which happen when substances are burned
in the air. The knowledge which was gained of the changes of
composition and of properties during combustion incited and
guided chemists to a searching examination of the distinctive
properties of many different substances, and this examination
brought about the clarifying of the conception of definite kinds
of matter, and the application of this conception to the opening of
many paths of chemical enquiry. While these advances were being
made, a quiet member of the Society of Friends presented chemistry
with a marvellously delicate and penetrative instrument for further-
ing accurate knowledge of material changes. John Dalton made
## p. 272 (#302) ############################################
272
The Literature of Science [CH.
what seemed a small addition to the Greek atomic theory, an
addition which changed an interesting speculation into a scientific
theory. As the century went on, chemists began to elucidate
the connections between chemical events and physical phenomena.
The science of physical chemistry began.
Among those who investigated the phenomena of combustion
in the eighteenth, and early nineteenth, century, Priestley and
Cavendish are pre-eminent. Black was the first chemist to
make an accurate, quantitative examination of a particular,
limited, chemical change, and, by so doing, to give clearness to
the expression 'a homogeneous substance. ' The atomic theory
was Dalton's gift to science. From the many chemists who
amplified the work of Dalton, and used the conceptions of atom
and molecule to connect and explain new classes of chemical facts,
Williamson and Frankland may be selected as the representatives.
As workers in the borderland between chemistry and physics,
Graham and Faraday are specially to be remembered. The in-
vestigations of Davy touched and illuminated every side of
chemical progress.
Besides these men, who greatly enriched and advanced the
science of chemistry in the period under review, there were
many workers whose contributions cannot be considered here,
References are given in the bibliography to the writings of some
of them.
Joseph Priestley was a man of many gifts and a very versatile
mind. When a youth at an academy, he tells us that he 'saw
reason to embrace what is generally called the heterodox side of
almost every question. When about twenty-eight years of age,
he taught, in a school at Warrington, languages (he had a great
natural gift of tongues), oratory and criticism, elocution, logic,
natural phenomena, civil law and anatomy.
In the seventies of the eighteenth century, Priestley turned his
attention to different kinds of airs. He obtained and partially
examined many gases, but rarely troubled about separating them
completely from impurities. In August 1774, Priestley obtained a
large lens with which he concentrated the sun's rays on whatever
substance happened to come to his hand, with the object of finding
what air could be extracted from it. When he thus heated mer-
curius calcinatus per se (now called oxide of mercury), he obtained
an air in which a candle burned with a remarkably vigorous
flame. ' This result, he says, 'surprised me more than I can well
express. ' The new air was subjected to many tests; it always
6
## p. 273 (#303) ############################################
VIII] Priestley's Work on Gases
273
behaved in a very unexpected manner. He placed a mouse in his
new air; the mouse remained lively, and the air did not become
noxious. ' The results of other experiments caused Priestley
to lie awake through the night ‘in utter astonishment. ' At last,
he concluded that the new air was 'between four and five times as
good as common air. He regarded the new air as a very superior
kind of common air.
Priestley thought alchemically, not as a chemist. To the
alchemist, the properties of things were external wrappings which
might be removed from one thing and put round another, without
affecting the essential substance of either thing, which substance
it was the business of properties to hide from the uninitiated.
Priestley thought of different airs as identical, or nearly identical,
in substance, and only apparently different because of superficial
differences in the mantles, the properties, by which the essential
substance was concealed. When he obtained the air from burnt
mercury, he thought he had removed from common air something
which made it ‘noxious, vitiated, depraved, corrupt. ' He had not
learnt, what Black’s experiments, made twenty years before 1774,
might have taught him, that each particular, material thing is
known only by its properties. Priestley's forced explanation of the
facts which he himself discovered helped to convince investigators
that the notion of identity of substance hidden under differences
of properties is a great hindrance to the acquirement of accurate
knowledge of natural events.
Priestley could not get over his astonishment at the behaviour
of the new air. In science, one does well to be astonished; but,
to astonishment one must add investigation, to investigation,
reasoning, and, to reasoning, more investigation. Stopping at
astonishment, Priestley made his facts square with the theory that
dominated him, the theory of phlogiston. The phlogisteans taught
that something, which they had named phlogiston, the principle of
fire, rushes out of a burning substance as it burns. Phlogiston
was never captured. Priestley held that the elusive phlogiston is
a great corrupter of your airs or gases. He supposed that he had
deprived common air of this depraving principle; he named his
new gas dephlogisticated air. He invented many very ingenious
hypotheses to account for facts observed by himself. Had he
made a few accurate quantitative experiments, he might have
broken the toils of his favourite theory.
The French chemist Lavoisier saw the importance of Priestley's
discovery of dephlogisticated air, and, by a series of rigidly
18
E. L. XIV.
CH. VIII.
## p. 274 (#304) ############################################
274 The Literature of Science [ch.
>
quantitative experiments with tin and mercury, proved that, when
a substance burns in air, it combines with a constituent of the air,
which air-constituent is the gas prepared by Priestley. Lavoisier
called this gas oxygen, because many of its compounds are
acids.
Priestley's insatiable curiosity, his mental alertness, his im.
patience of details, were required for the advancement of chemistry,
no less than the passionless determination and the scrupulous
accuracy of Cavendish.
Henry Cavendish, of Peterhouse, was bred in the theory of
phlogiston, as Priestley was, and remained faithful to that theory,
as Priestley did. He thought of many airs, or gases, as more or less
phlogisticated forms of a few particular substances. Cavendish
described the explosion of a mixture of common air and inflammable
air (obtained by the action of acids on zinc) as one of the ways of
phlogisticating air. This process is accompanied by a decrease in
the volume of the interacting gases. Cavendish tried to discover
the cause of this decrease. He exploded accurately measured
volumes of dephlogisticated air (oxygen) and inflammable air
(hydrogen), and found that water was the sole product of the
change when dephlogisticated air was mixed with twice its volume
of inflammable air. The explanation which Cavendish gave of
this fundamentally important fact was confused and vague, because
he insisted on making the facts uphold the phlogistic theory.
Without knowing exactly what he had done, Cavendish had
determined the quantitative volumetric composition of water.
When the phlogistic theory had been swept away, the very
great importance of the accurate work of Cavendish became
manifest.
Joseph Black graduated as doctor of medicine in the university
of Edinburgh in 1755, presenting a thesis entitled Magnesia
alba, Quicklime, and other alcaline substances. That thesis is
probably the earliest example of a genuinely scientific chemical
investigation. Black proved that mild magnesia (now called
magnesium carbonate) loses weight when it is calcined; he deter-
mined the loss of weight; he proved that the solid substance
which remains after calcination has properties of its own which
distinguish it from mild magnesia; he showed that, during calci-
nation, an air, or gas, is given off, different from any air, or gas,
then known ; he examined, accurately, the properties of this gas,
which he called fixed air; and he reproduced the original quantity
of mild magnesia by dissolving the calcined magnesia in acid, and
>
## p. 275 (#305) ############################################
VIII) Black and Cavendish
275
adding fixed alkali (now called potassium carbonate), a substance
which he proved to give off fixed air when it is calcined. By his
experiments, Black proved mild magnesia to be composed of fixed
air united with calcined magnesia, and showed that each of these
three substances is a particular and definite kind of matter, dis-
tinguished from all other kinds of matter by constant qualities.
He also proved that the change which happens when chalk is
burned is exactly similar to the calcining of mild magnesia ; fixed
air is driven out of the chalk, and burnt lime—a perfectly
definite homogeneous substance-remains.
The work of Black prepared the way for the penetrative, ex-
perimental analysis of the phenomena of combustion ; it taught
chemists to use accurately observed properties of bodies as the
only means of distinguishing one body from another; it showed
that, if chemical investigation is to produce results of permanent
value, it must be quantitative; incidentally, by isolating and
examining fixed air, it began a new branch of chemistry, the study
of the changes of composition and properties which happen when
homogeneous gases interact.
Black and Cavendish were painstaking, methodical, un-
emotional, eminently clear-headed. Priestley was flighty, fitting
from one thing to another in his laboratory, always curious, never
working out his discoveries, unable to think chemically outside of
the theory which dominated him. Black, Cavendish and Priestley
greatly advanced the science of chemistry.
So long as chemists formed vague generalisations founded on
introspective speculations, they made little progress. It was by
concentrating their attention on a few limited occurrences, and
accurately examining these by quantitative experiments, that
chemists gradually gained clear conceptions which could be
directly used in the investigation of more complicated chemical
changes. “True genius,' Coleridge said, 'begins by generalising
and condensing; it ends in realising and expanding. ' The vague
generalising of the alchemists was followed by the condensing
work of Black and Cavendish, and by the suggestive discoveries of
Priestley. The time was approaching for realising and expanding.
In 1808, a small book appeared, entitled A new system of
Chemical Philosophy, Part I, by John Dalton. The influence of
that book on the development of chemistry, and of physics also,
has been very great.
Dalton delivered a lecture in Manchester, in 1803, wherein he
said 'An enquiry into the relative weights of the ultimate particles
18-2
## p. 276 (#306) ############################################
276
The Literature of Science [CH.
of bodies is a subject, so far as I know, entirely new; I have lately
been prosecuting this enquiry with remarkable success. ' Many of
Dalton's predecessors, both chemists and physicists, had used, in a
vague and general manner, the Greek conception of the atomic
structure of matter. Dalton showed how the relative weights of
atoms can be determined. By doing that, he brought down the
atomic theory to the solid earth, and made it a bold, suggestive,
stimulating guide ready for the use of chemists and physicists.
Dalton was not a great experimenter; he generally used the
results of other chemists' experiments. He was a scientific thinker,
characterised by boldness and caution. Dalton assumed, as
Lucretius had done long before him, that matter has a grained
structure ; that all the ultimate particles of each particular
homogeneous substance are identical, and differ in properties,
one of which is their weight, from the particles of all other
definite substances; he also assumed that the mechanism of
chemical changes, that is, changes wherein homogeneous substances
are produced different from those present when the changes began,
is the coalescence of atoms of different kinds to form new sorts of
atoms.
In order to find the relative weights of atoms, Dalton argued
as follows: Analyses and syntheses of water show that eight
grains of oxygen unite with one grain of hydrogen to form water.
If this change is the union of atoms of oxygen with atoms of
hydrogen, to form atoms of water, and if all the atoms of each
one of these three homogeneous substances are identical in weight
and other properties, it follows that an atom of oxygen is eight
times heavier than an atom of hydrogen. If we take the atomic
weight of hydrogen as unity—because hydrogen is lighter than
any other known substance—then the atomic weight of oxygen is
eight, and the atomic weight of water is nine.
In arriving at the conclusion that the atomic weight of oxygen
is eight, if the atomic weight of hydrogen is one, Dalton made the
assumption that a single atom of oxygen unites with one atom of
hydrogen to form an atom of water. He made this assumption
because it was simpler than any other. Had he chosen to suppose
that two atoms of hydrogen unite with one atom of oxygen, he
must have assigned to oxygen the atomic weight sixteen, and to
water the atomic weight eighteen.
To make Dalton's method perfectly general, and quite conclusive
in its results, it was necessary to find means for fixing the relative
weights of the atoms formed by the union of other, simpler, atoms;
## p. 277 (#307) ############################################
VII] The Atomic Theory. Dalton and Others 277
it was also necessary to find means of determining the number of
atoms of each kind which unite to form a more complex atom.
A general method for solving these two problems was given to
chemistry in 1811–12 by an Italian physical chemist named
Avogadro, who brought into science the notion of a second order
of minute particles, supplementing the conception of atom by that
of molecule.
It is not possible in this brief sketch to indicate the many new
fields of investigation which were opened, and made fruitful, by
the Daltonian atomic theory. From the many workers who used
this theory as a means for pressing forward along new lines of
enquiry, two may be selected, since their work is typical of much
that was done in chemistry during the first half of the nineteenth
century.
Alexander Williamson strove to make chemists realise the need
of using the Avogadrean molecule as well as the Daltonian atom.
By his work on etherification, and by other experimental investiga-
tions, as well as by reasoning on his own results and those obtained
by other chemists, Williamson demonstrated the fruitfulness of
the notion of the molecule. He endeavoured to determine the
relative weights of molecules by purely chemical methods. These
methods proved to be less satisfactory, and much less general,
than the physical method which had been described by Avogadro.
The conception of equivalency, that is, equal value in exchange,
of determinate weights of different homogeneous substances, has
been very helpful in chemistry.
In 1852, Edward Frankland
applied the notion of equivalency to the atoms of elements, that
is, homogeneous substances which have not been separated into
unlike parts. He arranged the elements in groups, the atoms of
those in any one group being of equal value in exchange, inasmuch
as each of these atoms combines with the same number of other
atoms to form molecules.
When Frankland's conception had been developed, and the
method of determining the equivalency of atoms made more
definite and more workable, a vast new field of enquiry was
opened, a eld which has proved remarkably fruitful both in
purely scientific work, and in applied chemistry. It is not an
exaggeration to say that the great industry of making aniline
colours is an outcome of the notion of atomic equivalency intro-
duced by Frankland into chemical science.
The words element and principle were used by the alchemist
as nearly synonymous; both words were used vaguely. The
## p. 278 (#308) ############################################
278
[ch.
The Literature of Science
meaning given to the term element, by Lavoisier, towards the
end of the eighteenth century—a definite kind of matter which
has not been decomposed, that is, separated into unlike parts-
was elucidated, and confirmed as the only fruitful connotation
of the term by the work of Sir Humphry Davy on potash and soda
in 1808.
Humphry Davy was the most brilliant of English chemists.
He was the friend of Wordsworth and Sir Walter Scott. Lockhart
says that the conversation of Davy and Scott was fascinating and
invigorating. Each drew out the powers of the other.
6
I remember William Laidlaw whispering to me, one night when their
‘rapt talk 'had kept the circle round the fire until long after the usual bedtime
of Abbotsford-Gude preserve us! this is a very superior occasion 1! '
Davy sent an electric current through pieces of potash and soda;
the solids melted, and 'small globules, having a high metallic
lustre, and being precisely similar in visible characters to quick-
silver, appeared. By burning the metal-like globules, Davy
'
obtained potash and soda. Making his experiments quantitative,
weighing the potash and the soda before passing the current, and
the potash and soda obtained by burning the metal-like products
of the first change, he proved that potash and soda, which, at that
time, were classed with the elements, are composed each of a metal
combined with oxygen. The new metals—potassium and sodium-
are soft and very light, and instantly combine with oxygen when
they are exposed to the air.
Everyone had been accustomed to think of a metal as a heavy,
hard solid, unchanged, or very slowly changed, by exposure to air.
Had chemists strictly defined the term metal, they could not have
allowed the bases of potash and soda (as Davy called the new sub-
stances) to be included among metals. Happily, the definitions of
natural science are not as the definitions of the logician; they are
descriptive summaries of what is known, and suggestive guides to
further enquiry.
As every attempt to separate potassium and sodium into unlike
parts failed, Davy put them into the class elements ; he said—Till
a body is decomposed, it should be considered as simple. '
In 1810, Davy investigated a substance concerning the
composition of which a fierce controversy raged. Oxymuriatic
acid was said by almost all chemists at that time to be a compound
of oxygen with an unknown base. No one had been able to get
1 Life of Sir Walter Scott (6 vols. , 1900), vol. III, p. 403.
## p. 279 (#309) ############################################
a
6
VII] Davy. Physical Chemistry
279
oxygen from it, or to isolate the base supposed to be a constituent
of it. By putting away, for the time, all hypotheses and specula-
tions, and by conducting his experiments quantitatively, Davy
showed that oxymuriatic acid is not an acid, but is a simple
substance, that is, a substance which is not decomposed in any
of the changes it undergoes. He proposed to name this simple
substance chlorine ; a name, Davy said, 'founded upon one of its
obvious and characteristic properties-its colour. ' Davy re-
marked— Names should express things not opinions. '
Davy thought much about the connections between chemical
affinity and electrical energy, and investigated these connections
by well planned experiments. In 1807, he said—May not the
electrical energy be identical with chemical affinity ? ' He used
the expressions—'different electrical states,' and 'degrees of
exaltation of the electrical states,' of the particles of bodies.
Recent researches into the subject of chemical affinity have
established the great importance of the conceptions adumbrated
by Davy in these expressions.
Chemistry, the study of the changes of composition and
properties which happen when homogeneous substances interact,
has always been closely connected with physics, the study of the
behaviour of substances apart from those interactions of them
in which composition is changed. Among the earlier physical
chemists, Graham occupies an important place.
Thomas Graham was a shy, retiring man, most of whose life
was spent in his laboratory. There is a tradition in the Glasgow
institution, where he taught chemistry, in his younger days, before
moving to London (in his later years he was master of the mint),
that, when he came into the lecture theatre, to deliver his first
lecture to a large audience, he looked around in dismay and fled.
Graham established the fundamental phenomena of the diffusion
of gases and of liquids; he distinguished, and applied the distinction,
between crystalloids, solutions of which pass through animal and
vegetable membranes, and colloids, which do not pass through
those membranes. The investigation of the behaviour of colloidal
substances has led, in recent years, to great advances in the
knowledge of phenomena common to chemistry, physics and
biology.
Electrochemistry, the study of the connections between chemical
and electrical actions, has been productive, in recent years, of more
far-reaching results than have been obtained in any other branch
of physical chemistry. Much of what has been done in the last
a
## p. 280 (#310) ############################################
280 The Literature of Science [CH.
half-century is based on the work of Faraday, and, indirectly, on the
suggestion of Davy. Both were men of genius, that is, men who
see the central position of the problem they are investigating, who
seize and hold that position until the problem is solved, letting the
surface phenomena, for the time, go to the dogs, what matters? '
Men of genius work from the centre outwards.
To Michael Faraday, we owe the fundamental terms of electro-
chemistry. The separation of a salt into two parts by the electric
current, he called electrolysis ; the surfaces from which the current
passes into, and out of, an electrolysable compound, he named
electrodes; the substances liberated at the electrodes, he called
ions. Faraday measured the chemical power of a current' by
the quantities of the ions set free during a determinate period
of electrolysis. Taking as his unit the quantity of electricity
which liberates one gram of hydrogen from an electrolysable
compound of that element, he showed that the weights of different
ions liberated from compounds by unit quantity of electricity are
in the proportion of their chemical equivalents. Using the language
of the atomic theory, Faraday declared that 'the atoms of bodies
which are equivalent to each other in their ordinary chemical action
have equal quantities of electricity mutually associated with them. '
In 1834, Faraday said— The forces called electricity and
chemical affinity are one and the same. ' Faraday distinguished
the intensity of electricity from the quantity of it, and indicated
the meaning of each of these factors. One would not greatly
exaggerate if one said that the notable advances made in the
last quarter of a century in the elucidation of chemical affinity
are but developments and applications of Faraday's pregnant
work on the two factors of electrical energy.
The results established by Faraday have led to the conception
of atoms of electricity, a conception which has been of great
service in advancing the study of radioactivity. Faraday's results
have also been the incentives and guides in researches which go
to the root of many problems of the physical sciences, and of not
a few of the biological sciences also.
At the time of the foundation of the Royal Society, chemistry
was a conglomeration of more or less useful recipes, and a dream
of the elixir. Today, chemistry is becoming an almost universal
science. Happily, chemists still dream.
## p. 281 (#311) ############################################
VIII]
Biology
281
C. BIOLOGY
6
Although science, during the eighteenth century, was, like
many other intellectual activities in our country, more or less
in abeyance, an attempt has been made, in the following pages,
to carry on the subject in the present chapter from that which
appeared in a previous volume (VIII) of this History.
'The Royal Society of London for Improving Natural Know-
ledge, one of the oldest scientific societies in the world and
certainly the oldest in the empire, was formally founded in 1660,
and received its royal charter of incorporation two years later.
At a preliminary meeting, a list had been prepared of some forty
names of such persons as were known to those present whom
they judged willing and fit to joyne. . . in the designe,' and among
these names we find those of 'Mr Robert Boyle, Sir Kenelme
Digby, Mr Evelyn, Dr Ward, Dr Wallis, Dr Glisson, Dr Ent,
Dr Cowley, Dr Willis, Dr Wren' names whose owners have been
dwelt upon in volume VIII.
Thus, for the first time in our country, the study of science was,
to a degree, organised and its advancement promoted, not only
by periodical meetings where experiments were conducted and
criticism freely offered, but by the collection of scientific books,
which still remain at Burlington house, and of 'natural objects,
which have for long formed part of the British Museum's
collections.
So Virtuous and so Noble a Design,
So Human for its Use, for Knowledge so Divine,
as Abraham Cowley, the laureate of the new movement, wrote,
was, in part, a protest against the credulity and superstitions of
a credulous and superstitious age, and the word ‘natural, as
used in the charter, was used in deliberate opposition to “super-
natural,' the aim of the society being, at any rate in part, to
discourage divination and witchcraft.
We have said something about the brilliant band of physio-
logists, headed by Harvey, who made the Stewart period remarkable
in the annals of English science; though there were then other
biologists less gifted than Harvey, but still leaders in their several
fields. The recent invention of the microscope had given a great
impetus to the study of the anatomical structure of plants and,
later, of animals; and, in relation to this, we must not overlook
## p. 282 (#312) ############################################
282 The Literature of Science [CH.
the work of Nehemiah Grew, who, with the Italian Malpighi, may
be considered a co-founder of the science of plant-anatomy.
Nehemiah Grew studied at Pembroke hall, Cambridge, and after-
wards took his doctor's degree at Leyden. He published numerous
treatises dealing with the anatomy of vegetables, and with the
comparative anatomy of trunks, roots, and so forth, illustrated
by admirable, if somewhat diagrammatic, plates. Although
essentially an anatomist, he made certain investigations into
plant physiology and suggested many more. Perhaps his most
interesting contribution to botany, however, was his discovery
that flowering plants, like animals, have male and female sexes.
It seems odd to reflect that this discovery is only about 250 years
old. When Grew began to work, the study of botany was in a
very neglected condition—the old herbal had ceased to interest,
and, with its contemporary, the bestiary, was disappearing from
current use, while the work of some of Grew's contemporaries,
notably Robert Morison and John Ray, hastened their dis-
appearance. Of these two systematists, Ray, on the whole, was the
more successful. His classification of plants obtained in England
until the latter half of the eighteenth century, when it was gradually
replaced by the Linnaean method of classification.
But Ray has other claims on our regard. He and Francis
Willughby, both of Trinity college, attacked a similar problem in
the animal kingdom. Willughby was the only son of wealthy and
titled parents, while Ray was the son of a village blacksmith.
But the older universities are great levellers, and Ray succeeded
in infusing into his fellow student at Cambridge his own genuine
love for natural history. With Willughby, he started forth on his
methodical investigations of animals and plants in all the accessible
parts of the world. Willughby died young and bequeathed a
small benefaction and his manuscripts to his older friend. After
his death, Ray undertook to revise and complete his Ornithology,
and therein paid great attention to the internal anatomy, to the
habits and to the eggs of most of the birds he described. He, further,
edited Willughby's History of Fishes, but perpetuated the mistake
of his predecessors in retaining whales among that group.
In
rather rationalistic mood, he argues that the fish which swallowed
Jonah must have been a shark. Perhaps the weakest of their
three great histories—the History of Insects—was such owing
to the fact that Ray edited it in his old age.
Ray was always a fine field naturalist, and his catalogues of
Cambridgeshire plants long remained a classic. We may, perhaps,
## p. 283 (#313) ############################################
VIII] Ray, Willughby and Hooke 283
sum up the contributions of this great naturalist in the words of
Miall :
During his long and strenuous life he introduced many lasting improve-
ments-fuller descriptions, better definitions, better associations, better
sequences. He strove to rest his distinctions upon knowledge of structure,
which he personally investigated at every opportunity. . . . His greatest
single improvement was the division of the herbs into Monocotyledons and
Dicotyledons 1
Robert Hooke, a Westminster boy and, later, a student at
Christ Church, was at once instructor and assistant to Boyle.
The year that the Royal Society received their charter, they
appointed Hooke curator, and his duty was 'to furnish the
Society' every day they met with three or four considerable
experiments. This amazing task he fulfilled in spite of the fact
that the fabrication of instruments for experiments was not
commonly known to workmen,' and that he never received above
£50 a year and that not certain. ' Hooke was a man of amazing
versatility, very self-confident, attacking problems in all branches
of science, greatly aiding their advance, but avid of fame.
In person but dispicable, being crooked and low in nature and as he grew
older more and more deformed. He was always very pale and lean and
latterly nothing but skin and bone 2.
His active, jealous mind conceived that almost every discovery of
his time had been there initiated; and this anxiety to claim ‘priority'
induced Newton to suppress his treatise Optics until after the date
of Hooke's death. His book Micrographia, 'a most excellent
piece, of which I am very proud,' as Pepys has it, is the record of
what a modern schoolboy newly introduced to the microscope would
write down. Yet he was undoubtedly, although not a lovable
character, the best 'mechanic of his age. '
British physiology, which had started magnificently with
Harvey, and had continued under Mayow, de Mayerne and others,
was carried forward by Stephen Hales, at one time fellow of
Corpus Christi college, Cambridge, and for years perpetual curate
at Teddington. He was a born experimenter, and, as a student,
worked in the 'elaboratory of Trinity College, which had been
established under the rule of Bentley, ever anxious to make his
college the leader in every kind of learning. Sachs has pointed
out that, during the eighteenth century, the study of the anatomy
of plants made but little progress ; but there was a very real
1 The Early Naturalists, L. C. Miall, London, 1912.
? Waller's Life of Hooke, 1705.
## p. 284 (#314) ############################################
284 The Literature of Science [CH.
advance in our knowledge of plant physiology. This, in the main,
was due to Hales; he investigated the rate of transpiration and
held views as to the force causing the ascent of sap which have
recently come to their own; he recognised that the air might
be a source of food for the plant and connected the assimilative
function of leaves with the action of light,' though he failed to
find the mode of the interaction. He worked much on gases, and
paved the way for Priestley and others by devising methods of
collecting them over water. Hales, this 'poor, good, primitive
creature,' as Horace Walpole called him, was not less remarkable
as an investigator of animal physiology, and was the first to
measure the blood-pressure, and the rate of flow in the capillaries.
Sir Francis Darwin states:
In first opening the way to a correct appreciation of blood-pressure Hales'
work may rank second in importance to Harvey's in founding the modern
science of physiology.
He was, further, a man of 'many inventions,' especially in the fields
of ventilation and hygiene.
The beginning of our period coincides with the formation of
public museums. Previous to the Stewart times, collections of
‘natural objects' were, for the most part, housed in churches, in the
houses of the great, in coffee-houses and in the shops of apothe-
caries; but now public libraries were being established, and, in many
of these, botanical, geological and especially zoological specimens
found a home. In more than one Cambridge college, the library
still gives shelter to a skeleton, a relic of the time when anatomy
was taught within the college walls; and, at this day, the curious,
and, at times, inconvenient, yoke joining the museum at South
Kensington with the museum in Bloomsbury testifies to this
primitive state of affairs.
In 1728, John Woodward bequeathed his 'Fossils, a vast quan-
tities of Ores, Minerals and Shells, with other curiosities well
worth viewing' to Cambridge university; it was housed in the
university library and formed the nucleus about which the present
magnificent museum has collected. For many years, the Royal
Society maintained a museum which, at one time, contained 'the
stones taken out of Lord Belcarre's heart in a silver box,'. . .
‘a petrified fish, the skin of an antelope which died in St James'
Park, a petrified foetus' and 'a bottle full of stag's tears. ' The
trustees of Gresham college assigned the long gallery as a home
for these and other 'rarities'; but, when the society, in 1781,
migrated to Somerset house, the entire collection was handed over
## p. 285 (#315) ############################################
VIII] Origin of Museums
285
to the British Museum. The charter of the last named is dated
1753, and its beginnings were the library of Sir Robert Cotton,
wbich the nation had purchased in 1700, and the collections of
Sir Hans Sloane, which were now purchased with the proceeds of
a lottery, set on foot for this purpose. The collections of this
General Repository,' as the act of 1753 called the museum, were
kept together until the middle of the nineteenth century, when,
after long delay, the natural history objects were transferred to
South Kensington and housed in a building which, in all respects,
was worthy of the Board of Works of the time.
John Tradescant and his son of the same name accumulated
and stored in south Lambeth a 'museum which was considered to
be the most extensive in Europe at that time. It was acquired in
1659 by Elias Ashmole, and, with his own collections, passed
by gift, twenty-three years later, to Oxford university, the whole
forming the nucleus of the present Ashmolean museum.
Want of space precludes the consideration of other museums;
but it may be remarked that the earlier collectors got together
their treasures much as schoolboys now collect, their taste was
universal and no rarity was too trivial for their notice. Such collec-
tions excited popular interest, and 'a museum of curiosities' was
often an added attraction to the London coffee-house. At the end of
the eighteenth century, the coffee-house part of the enterprise was
dropped, and the museum, with an entrance-fee and a priced cata-
logue, formed a source of revenue to many a collector, most
of whom were not too scrupulous in their identifications. The
dime museums in the Bowery, New York, are their modern
successors. These museums were of little scientific or educational
value ; at best, they stimulated the imagination of the uninformed,
or allowed a child to see with his own eyes something he had read
about in his books. The normal, as a rule, was passed by, the
abnormal treasured. Ethnographical objects were collected, not
so much to arouse in the spectator a desire to study seriously
‘y® beastlie devices of yo heathen' as to excite and startle him
with their rough unfinish, on the one hand, and their high finish
on the other. The collections of the museums were ill arranged,
inaccurately labelled and inaccessible to students; the staff
were wholly inadequate and mainly dependent for their living
on admission fees. It was not until the nineteenth century
was well advanced that a systematic and scientific attempt
was made to identify specimens accurately, to arrange them
logically, to label them fully and, further, to collect in the
6
## p. 286 (#316) ############################################
286 The Literature of Science [CH.
background, unseen by the fleeting visitor, vast accumulations
of material for the investigation of the genuine student and
researcher.
Museums as centres of real education, not as places of wonder
and vacant amazement, are almost affairs of our time, and it
was not until the twentieth century that official guides were
appointed to explain their treasures to the enquiring visitor.
Even today, the system of weekly lectures on the contents of a
museum which obtains largely on the other side of the Atlantic
is, with us, only beginning.
We must not omit to mention the magnificent museum of the
Royal college of Surgeons, in London, which incorporates the
Hunterian collection brought together by John Hunter, and which
has been growing ever since his time. Of its kind, it is without a
rival in the world.
During the seventeenth century, men of science still, to a
great extent, remained the gifted amateurs they were at the time
of the foundation of the Royal Society; and yet they were very
successful in establishing many institutions which had a greater
effect on the advance of biological sciences than their founders
foresaw.
Towards the beginning of the seventeenth century, the Oxford
botanic garden had been founded (1621), which was followed, in
1667, by the opening of the Edinburgh botanic garden, and, in 1673,
by the foundation of the Chelsea physic garden, by the Apothe-
caries' company.
At the beginning of the eighteenth century,
Glasgow followed suit. By this time, many of the universities had
chairs of botany, and botany and anatomy were the first biological
sciences represented by professorial chairs in this country. In
1724, a chair was established at Cambridge, with Bradley as
its first professor ; but he and his immediate followers had little
success and, for the most part, were non-resident. Oxford
followed, in 1734, and Dillenius was the first to occupy the chair,
which had been founded by William Sherrard. The botanic
garden at Oxford, however, had been in existence for many years.
At Cambridge, it was not till 1759 that Walker founded the
botanic garden, which, at that time, occupied the northern site
of the present museums of science. The fine specimen of the
Sophora tree, the tree which yields the Chinese imperial yellow
dye, is the last and only memorial of this old botanic garden.
In 1765, Kew gardens, originally in possession of the Capel family,
were combined with Richmond gardens, then occupied by the
## p. 287 (#317) ############################################
viii] Botanic Gardens and Learned Societies 287
princess Augusta, widow of Frederick, prince of Wales. In fact,
this lady may be regarded as the foundress of Kew, which, since
her time, has played the leading part in the dissemination of
botanical knowledge throughout the world.
In the latter half of the eighteenth century, the Linnaean
system of classification had been generally adopted in Great
Britain, and, in the year 1783, Sir James Edward Smith secured,
from the mother of Linnaeus, for £1050, the entire Linnaean
collections. These did not, however, reach these islands without
an effort on the part of the Swedish government to retrieve
them. Indeed, it sent a man-of-war after the ship which
transported them.
Following on this acquisition, Smith, in 1788, founded the
Linnaean society, the immediate effect of which, perhaps, was
to bring about a revolution in the mode of publishing scien-
tific literature From the first, the Linnaean society issued
journals and transactions instead of books or treatises; their
publications took the form of memoirs read before the society.
In this respect, the Linnaean society set a fashion which has been
consistently followed by the numerous societies which since have
sprung up.
The Royal Society had taken all science as its province, and
nothing in natural history was alien to the activities of the
Linnaean society ; but, with the beginning of the nineteenth
century, societies began to spring up in the metropolis which
devoted their energies to the advancement of one science alone.
The earliest effort was that of the Royal Horticultural society,
founded in 1803. Its first secretary was Joseph Sabine, to whom
much of its earlier success was due. For a time, it undertook
the training of gardeners and also sent collectors to foreign
countries in search of horticultural rarities. It still does much
for horticulture, especially by its very successful flower-shows.
The Geological society of London was founded in 1807. It was
partly the outcome of a previous club known as the Askesian
society, and among the more prominent founders were William
Babington, Humphry Davy, George Greenough and others. The
meetings were at first held at the Freemasons' tavern. The
society, like many other learned societies, now has rooms at
Burlington house.
The Zoological society of London for the advancement of
zoology and animal physiology, and for the introduction of new
and curious subjects of the animal kingdom was founded in
## p. 288 (#318) ############################################
288
[ch.
The Literature of Science
1826 by Sir Stamford Raffles, the wellknown traveller and governor
in the east and the godfather of Rafflesia, J. Sabine, N. A. Vigors
and other eminent naturalists. It was incorporated by royal
charter in 1829.
The Royal Botanic society was founded in 1839, and was
granted an area of eighteen acres within the inner circle of
Regent's park, and here Marnock laid out the gardens very much
as they still are. Shortly after its establishment, annual exhi-
bitions or flower-shows were begun, and such exhibitions, not
entirely confined to flowers, are still one of the features of the
society.
Another society which has played a most useful part in the
promotion of science is the Cambridge Philosophical society,
founded in the year 1819, the only society outside the capital
towns which possesses a royal charter. About the same time,
the Dublin society (founded in 1731) assumed the title royal
The Edinburgh Royal society was founded in 1783; the date of its
revised charter is 1811. Many other societies in our chief towns
did much to advance the cause of science; but they are too
numerous to record here.
Another institution which embraced all branches of science was
the British Association for the Advancement of Science, which was
due largely to the enterprise of Brewster, Babbage and Herschel
It held its first meeting in York in the year 1831. The objects of
its founders were
to give a stronger impulse and a more systematic direction to scientifie
enquiry, to promote the intercourse of those who cultivate science in different
parts of the British Empire with one another, and with foreign philosophers,
to obtain a more general attention the objects of science, and the removal
of any disadvantages of a public kind, which impede its progress.
With certain exceptions, the books on biology during the last
half of the eighteenth century and the beginning of the nineteenth,
were largely treatises on classification, or on the practical applica-
tion of the knowledge of plants, such as medical and agricultural
works. It was during this period, too, that certain magazines
were started. Curtis founded The Botanical Magazine in the
year 1787. But the great increase of scientific journals only
began some fifty years later; many of those dealing with different
branches of biological science were first published about the
middle of the nineteenth century. Among them may be men-
tioned the following, with the date of their first appearance:
The Annals and Magazine of Natural History, 1841 ; The
## p. 289 (#319) ############################################
VIII] Scientific Journals and Exploration 289
Zoologist, 1843 ; Quarterly Journal of Microscopical Science,
1853; The Journal of Horticulture, 1862; The Geological
Magazine, 1864; The Journal of Anatomy and Physiology,
1866.
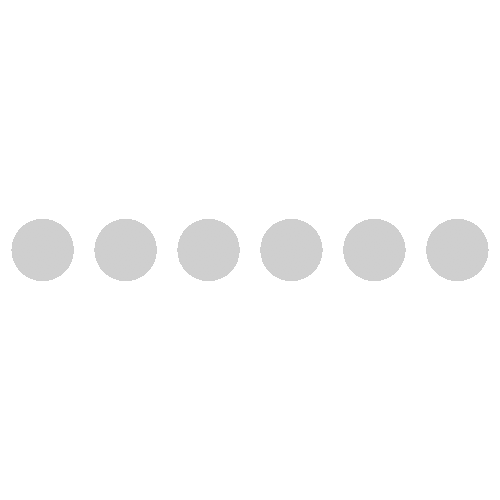