Lots of influences have contributed to computer
development
which are not going to help us to understand brains.
Richard-Dawkins-Unweaving-the-Rainbow
The brain puts the two together and sees an impressively three-dimensional scene.
Julesz did the same thing, except that his pictures were random pepper and salt dots.
The left and the right eye were shown the same random pattern, but with a crucial difference.
In a typical Julesz experiment, an area of the pattern, say, a square, has its random dots displaced to one side, the appropriate distance to create the stereoscopic illusion.
And the brain sees the illusion - a square patch stands out - even though there is not the smallest trace of a square in either of the two pictures.
The square is present only in the discrepancy between the two pictures.
The square looks very real to the viewer, but it really is nowhere but in the brain.
The Julesz Effect is the basis of the 'Magic Eye' illusions so popular today.
In a tour de force of the explainer's art, Steven Pinker devotes a small section of How the Mind Works (1998) to the
principle underlying these pictures. I won't even try to better his explanation.
There is an easy way to demonstrate that the brain works as a sophisticated virtual reality computer. First, look about you by moving your eyes. As you swivel your eyes, the images on your retinas move as if you were in an earthquake. But you don't see an earthquake. To you, the scene seems as steady as a rock. I am leading up, of course, to saying that the virtual model in your brain is constructed to remain steady. But there is more to the demonstration, because there's another way to make the image on your retina move. Gently poke your eyeball through the skin of the eyelid. The retinal image will move in the same kind of way as before. Indeed you could, given sufficient skill with your finger, mimic the effect of shifting your gaze. But now you really will think you see the earth move. The whole scene shifts, as if you were witnessing an earthquake.
What is the difference between these two cases? It is that the brain computer has been set up to take account of normal eye movements and make allowance for them in constructing its computed model of the world. Apparently the brain model makes use of information, not only from the eyes, but also from the instructions to move the eyes. Whenever the brain issues an order to the eye muscles to move the eye, a copy of that order is sent to the part of the brain that is constructing the internal model of the world. Then, when the eyes move, the virtual reality software of the brain is warned to expect the retinal images to move just the right amount, and it makes the model compensate. So the constructed model of the world is seen to stay still, although it may be viewed from another angle. If the earth moves at any time other than when the model is told to expect movement, the virtual model moves accordingly. This is fine, because there really might be an earthquake. Except that you can fool the system by poking your eyeball. As the final demonstration using yourself as guinea pig, make yourself giddy by spinning round and round. Now stand still and look fixedly at the world. It will appear to spin even though your reason tells you that it is not getting anywhere in its rotation. Your retinal images are not moving, but the accelerometers in your ears (which work by detecting the movements of fluid in the so-called semicircular canals) are telling the brain that you are spinning. The brain instructs the virtual reality software to expect to see the world spinning. When the images on the retina do not spin, therefore, the model registers the discrepancy and spins itself in the opposite direction. To put it in subjective language, the virtual reality software says to itself, 'I know I'm spinning from what the ears are telling me; therefore, in order to hold the model still, it will be necessary to put the opposite spin on the model, relative to the data that the eyes are sending in. ' But the retinas actually report no spin, so the compensating
spin of the model in the head is what you seem to see. In Barlow's terms, it is the unexpected, it is 'news', and that is why we see it.
Birds have an additional problem which humans ordinarily are spared. A bird perched on a tree branch is constantly being blown up and down, to and fro, and its retinal images seesaw accordingly. It is like living through a permanent earthquake. Birds keep their heads, and hence their view of the world, steady by diligent use of the neck muscles. If you film a bird on a windblown branch, you can almost imagine that the head is nailed to the background, while the neck muscles use the head as a fulcrum to move the rest of the body. When a bird walks, it employs the same trick to keep its perceived world steady. That is why walking chickens jerk their heads back and forth in what can seem to us quite a comical fashion. It is actually rather clever. As the body moves forward, the neck draws the head backwards in a controlled way so that the retinal images remain steady. Then the head shoots forward to allow the cycle to repeat. I can't help wondering whether, as an untoward consequence of the bird way of doing things, a bird might be unable to see a real earthquake because its neck muscles would automatically compensate. More seriously, we might say that the bird is using its neck muscles in a Barlow-style exercise: holding the non-newsworthy part of the world constant so that genuine movement stands out.
Insects and many other animals seem to have a similar habit of working to keep their visual world constant. Experimenters have demonstrated this in a so-called 'optomotor apparatus', where the insect is placed on a table and surrounded by a hollow cylinder painted on the inside with vertical stripes. If you now rotate the cylinder, the insect will use its legs to turn, keeping up with the cylinder. It is working to keep its visual world constant.
Normally, an insect has to tell its simulating software to expect movement when it walks, otherwise it would start compensating for its own movements, and then where would it be? This thought prompted two ingenious Germans, Erich von Hoist and Horst Mittelstaedt, to a diabolically cunning experiment. If you've ever watched a fly washing its face with its hands, you will know that flies are capable of flicking their head completely upside down. Von Hoist and Mittelstaedt succeeded in fixing a fly's head in the inverted position using glue. You have already guessed the consequence. Normally, whenever a fly turns its body, the model in its brain is told to expect a corresponding movement of the visual world. But as soon as it took a step, the wretched fly with its head upside down received data suggesting that the world had moved in the opposite direction to the one expected. It therefore moved its legs further in the same direction in order to compensate. This caused the apparent position of the world to move even further. The fly ended up spinning
round and round like a top, at ever-increasing speed - well, within obvious practical limits.
The same Erich von Hoist also pointed out that we should expect a similar confusion if our own voluntary instructions to move our eyes are neutralized, for example by narcotizing the eye-moving muscles. Normally, if you give your eyes the command to move to the right, your retinal images will signal a move to the left. To compensate and create the appearance of stability, the model in the head has to be moved to the right. But if the eye-moving muscles are narcotized, the model should move to the right in anticipation of what turns out to be a non-existent retinal movement. Let von Hoist himself take up the story, in his paper 'The Behavioural Physiology of Animals and Man' (1973):
This is indeed the case! It has been known for many years from people with paralysed eye muscles and it has been established exactly from the experiments of Kornmuller on himself that every intended but unfulfilled eye movement results in the perception of a quantitative movement of the surroundings in the same direction.
We are so used to living in our simulated world and it is kept so beautifully in synchrony with the real world that we don't realize it is a simulated world. It takes clever experiments like those of von Hoist and his colleagues to bring it home to us.
And it has its dark side. A brain that is good at simulating models in imagination is also, almost inevitably, in danger of self-delusion. How many of us as children have lain in bed, terrified because we thought we saw a ghost or a monstrous face staring in at the bedroom window, only to discover that it was a trick of the light? I've already discussed how eagerly our brain's simulation software will construct a solid face where the reality is a hollow face. It will just as eagerly make a ghostly face where the reality is a collection of moonlit folds in a white net curtain.
Every night of our lives we dream. Our simulation software sets up
worlds that do not exist; people, animals and places that never existed, perhaps never could exist. At the time, we experience these simulations as though they were reality. Why should we not, given that we habitually experience reality in the same way - as simulation models? The simulation software can delude us when we are awake, too. Illusions like the hollow face are in themselves harmless, and we understand how they work. But our simulation software can also, if we are drugged, or feverish, or fasting, produce hallucinations. Throughout history, people have seen visions of angels, saints and gods; and these have seemed very real to them. Well, of course they would seem real. They are models, put
together by the normal simulation software. The simulation software is
using the same modelling techniques as it uses ordinarily when it presents its continuously updated edition of reality. No wonder these visions have been so influential. No wonder they have changed people's lives. So if ever we hear a story that somebody has seen a vision, been visited by an archangel, or heard voices in the head, we should immediately be suspicious of taking it at face value. Remember that all our heads contain powerful and ultra-realistic simulation software. Our simulation software could knock up a ghost or a dragon or a saintly virgin in no time flat. It would be child's play for software of that sophistication.
A word of warning. The metaphor of virtual reality is beguiling and, in many ways, apt. But there is a danger of its misleading us into thinking that there is a 'little man' or 'homunculus' in the brain watching the virtual reality show. As philosophers such as Daniel Dennett have pointed out, you have explained precisely nothing if you suggest that the eye is wired to the brain in such a way that a little cinema screen, somewhere in the brain, continuously relays whatever is projected on the retina. Who looks at the screen? The question now raised is no smaller than the original question you think you have answered. You might as well let the little man look at the retina directly, which is clearly no solution to anything. The same problem arises if we take the virtual reality metaphor literally and imagine that some agent locked inside the head is 'experiencing' the virtual reality performance.
The problems raised by subjective consciousness are perhaps the most baffling in all philosophy, and solving them is far beyond my ambition. My suggestion is the more modest one that each species, in each situation, needs to deploy its information about the world in whatever way is most useful for taking action. 'Constructing a model in the head' is a helpful way to express how it is done, and comparing it to virtual reality is especially helpful in the case of humans. As I have argued before, the model of the world used by a bat is likely to be similar to the model used by a swallow, even though one is connected to the real world via the ears, the other via the eyes. The brain constructs its model world in the way most suited for action. Since the actions of day-flying swallows and night-flying bats are similar - navigating at high speed in three dimensions, avoiding solid obstacles and catching insects on the wing - they are likely to use the same models. I do not postulate a 'little bat in the head' or a 'little swallow in the head' to watch the model. Somehow the model is used to control the wing muscles, and that is as far as I go.
Nevertheless, each of us humans knows that the illusion of a single agent sitting somewhere in the middle of the brain is a powerful one. I suspect that the case may be parallel to the 'selfish Cooperator' model of
genes coming together, although they are fundamentally independent agents, to create the illusion of a unitary body. I'll briefly return to the idea near the end of the next chapter.
This chapter has developed the thesis that brains have taken over from DNA part of the role of recording the environment - environments, rather, for they are many and spread out over the near and the distant past. Having a record of the past is useful only in so far as it helps in predicting the future. The animal's body represents a kind of prediction that the future will resemble the ancestral past, in broad outline. The animal is likely to survive to the extent that this turns out to be true. And simulation models of the world allow the animal to act as if in anticipation of what that world is likely to throw its way in the next few seconds, hours or days. For completeness we must note that the brain itself, and its virtual reality software, are ultimately the products of natural selection of ancestral genes. We could say that the genes can predict a limited amount, because only in a general way will the future resemble the past. For the details and the subtleties, they provide the animal with nervous hardware and virtual reality software which will constantly update and revise its predictions to fit highspeed changes in circumstances. It is as if the genes say, 'We can model the basic shape of the environment, the things that don't change over the generations. But for the fast changes, over to you, brain. '
We move through a virtual world of our own brains' making. Our constructed models of rocks and of trees are a part of the environment in which we animals live, no less than the real rocks and trees that they represent. And, intriguingly, our virtual worlds must also be seen as part of the environment in which our genes are naturally selected. We have pictured camel genes as denizens of ancestral worlds, selected to survive in ancient deserts and even more ancient seas, selected to survive in companionship with compatible cartels of other camel genes. All that is true, and equivalent stories of Miocene trees and Pliocene savannahs can be told of our genes. What we must now add is that, among the worlds in which genes have survived are virtual worlds constructed inside ancestral brains.
In the case of highly social animals like ourselves and our ancestors, our virtual worlds are, at least in part, group constructions. Especially since the invention of language and the rise of artifact and technology, our genes have had to survive in complex and changing worlds for which the most economical description we can find is shared virtual reality. It is a startling thought that, just as genes can be said to survive in desserts or forests, and just as they can be said to survive in the company of other genes in the gene pool, genes can also be said to survive in the virtual,
even poetic worlds created by brains. It is to the enigma of the human brain itself that we turn in the final chapter.
12
THE BALLOON OF THE MIND
The brain is a three pound mass you can hold in your hand that can conceive of a universe a hundred billion light-years across.
MARIAN C. DIAMOND
It is a commonplace among historians of science that the biologists of any age, struggling to understand the workings of living bodies, make comparison with the advanced technology of their time. From clocks in the seventeenth century to dancing statues in the eighteenth, from Victorian heat engines to today's heat-seeking, electronically guided missiles, the engineering novelties of every age have refreshed the biological imagination. If, of all these innovations, the digital computer promises to overshadow its predecessors, the reason is simple. The computer is not just one machine. It can be swiftly reprogrammed to become any machine you like: calculator, word processor, card index, chess master, musical instrument, guess-your-weight machine, even, I regret to say, astrological soothsayer. It can simulate the weather, lemming population cycles, an ants' nest, satellite docking, or the city of Vancouver.
The brain of any animal has been described as its on-board computer. It does not work in the same way as an electronic computer. It is made
from very different components. These are individually much slower, but they work in huge parallel networks so that, by some means still only partly understood, their numbers compensate for their slower speed, and brains can, in certain respects, outperform digital computers. In any case, the differences of detailed working do not disempower the metaphor. The brain is the body's on-board computer, not because of how it works but because of what it does in the life of the animal. The resemblance of role extends to many parts of the animal's economy but, perhaps most spectacularly of all, the brain simulates the world with the equivalent of virtual reality software.
It might seem a good idea, in a general way, for any animal to grow a large brain. Isn't greater computing power always likely to be an advantage? Maybe, but it has costs, too. Weight for weight, brain tissue consumes more energy than other tissues. And our big brains as babies make it quite difficult for us to be born. Our presumption that braininess must be a good thing partly grows out of vanity in our species' own
hypertrophy of the brain. But it remains an interesting question why human brains have grown so especially big.
One authority has said that the evolution of the human brain over the last million years or so is 'perhaps tile fastest advance recorded for any complex organ in the entire history of life'. This may be an exaggeration, but the evolution of the human brain is undeniably fast. Compared with the skulls of other apes, the modern human skull, at least the bulbous part that houses the brain, has blown up like a balloon. When we ask why this happened, it is not satisfactory to produce general reasons why having a large brain might be useful. Presumably such general benefits would apply to many kinds of animal, especially those that navigate rapidly through the complicated three-dimensional world of the forest canopy, as most primates do. A satisfying explanation will be one that tells us why one particular lineage of apes - actually, one that had left the trees - suddenly took off, leaving the rest of the primates standing. It was once fashionable to lament - or, according to taste, gloat over - the paucity of fossils linking Homo sapiens to our ape ancestors. This has changed. We now have a rather good fossil series and as we go backwards in time we can trace a gradual shrinkage in braincase through various species of Homo to our predecessor genus Australopithecus whose braincase was about the same size as a modern chimpanzee's. The main difference between Lucy or Mrs Pies (famous Australopithecines) and a chimpanzee lay not in the brain at all, but in the Australopithecine habit of walking upright on two legs. Chimps only occasionally do. The blowing up of the brain balloon spanned three million years from Australopithecus through Homo habilis, then Homo erectus, through archaic Homo sapiens to modern Homo sapiens.
Something a bit similar seems to have happened in the growth of the computer. But, if the human brain has blown up like a balloon, the computer's progress has been more like an atom bomb. Moore's law states that the capacity of computers of a given physical size doubles every 1. 5 years. (This is a modern version of the law. When Moore originally stated it more than three decades ago he was referring to transistor counts which, on his measurements, doubled every two years. Computer performance has improved even faster because transistors became faster as well as smaller and cheaper. ) The late Christopher Evans, a computer-literate psychologist, put the point dramatically:
Today's car differs from those of the immediate post-war years on a number of counts. It is cheaper, allowing for the ravages of inflation, and it is more economical and efficient. . . But suppose for a moment that the automobile industry had developed at the same rate as computers and over the same period: how much cheaper and more efficient would the current models be? If you have not already heard the analogy the answer
is shattering. Today you would be able to buy a Rolls-Royce for ? 1. 35, it would do three million miles to the gallon, and it would deliver enough power to drive the Queen Elizabeth II. And if you were interested in miniaturization, you could place half a dozen of them on a pinhead. The Mighty Micro (1979)
Of course, things on the timescale of biological evolution inevitably happen far more slowly. One reason is that every improvement has to come about through individuals dying and rival individuals reproducing. So comparisons of absolute speed cannot be made. If we compare the brains of Australopithecus, Homo habilis, Homo erectus and Homo sapiens, we get a rough equivalent of Moore's law, slowed down by six orders of magnitude. From Lucy to Homo sapiens, brain size has approximately doubled every 1. 5 million years. Unlike Moore's law for computers, there is no particular reason to think that the human brain will go on swelling. In order for this to happen, large-brained individuals have to have more children than small-brained individuals. It isn't obvious that this is now happening. It must have happened during our ancestral past, otherwise our brains would not have grown as they did. It also must have been true, incidentally, that braininess in our ancestors was under genetic control. If it had not been, natural selection would have had nothing to work on, and the evolutionary growth of the brain would not have occurred. For some reason, many people take grave political offence at the suggestion that some individuals are genetically cleverer than others. But this must have been the case when our brains were evolving, and there is no reason to expect that facts will suddenly change to accommodate political sensitivities.
Lots of influences have contributed to computer development which are not going to help us to understand brains. A major step was the change from the valve (vacuum tube) to the much smaller transistor, and then the spectacular and continuing miniaturization of the transistor in integrated circuits. These advances are all irrelevant to brains, because - the point deserves repetition - brains don't work electronically anyway. But there is another source of computer advancement, and this might be relevant to brains. I'll call it self-feeding co-evolution.
We have already met co-evolution. It means the evolving together of different organisms (as in the arms races between predators and prey), or between different parts of the same organism (the special case called co- adaptation). As another example, there are some small flies whose appearance mimics that of a jumping spider, including large dummy eyes looking straight forward like paired headlights - very unlike the compound eyes with which the flies themselves see. Real spiders are potential predators of flies of this size, but they are put off by the flies' similarity to another spider. The flies enhance the mimicry by waving
their arms in ways that resemble the histrionic semaphore signals that jumping spiders use when courting their own opposite sex. In the fly, genes controlling the anatomical resemblance to spiders must have evolved together with separate genes controlling the semaphoring behaviour. This evolving together is co-adaptation.
Self-feeding is the name I am giving to any process in which 'the more
you have, the more you get'. A bomb is a good example. The atomic bomb is said to depend upon a chain reaction, but the metaphor of a chain is too stately to convey what happens. When the unstable nucleus of uranium 255 breaks up, energy is released. Neutrons shooting out from the break-up of one nucleus may hit another and induce it to break up
as well, but that is usually the end of the story. Most of the neutrons
miss other nuclei and shoot off harmlessly into empty space, for uranium, though one of the densest of metals, is 'really', like all matter, mostly empty space. (The virtual model of metal in our brains is constructed
with the persuasive illusion of dense solidity because that is the most useful internal representation of a solid for our survival purposes. ) On their own scale, the atomic nuclei in a metal are far more spaced out
than gnats in a swarm, and a particle expelled by one decaying atom is quite likely to have a clear run out of the swarm. If, however, you pack in a quantity (the famous 'critical mass') of uranium 235 which is just sufficient to see to it that a typical neutron expelled from any one
nucleus is on average likely to hit one other nucleus before leaving the mass of metal altogether, a so-called chain reaction gets going. On average, each nucleus that splits causes another to split, there is an epidemic of atom-splitting, with an exceedingly rapid release of heat and other destructive energy, and the results are only too well known. All explosions have this same epidemic quality find, on a slower time-scale, epidemics of disease sometimes resemble explosions. They require a critical mass of susceptible victims in order to get started and, once they do get started, the more you have the more you get. This is why it is so important to vaccinate a critical proportion of the population. If fewer than the 'critical mass' remain unvaccinated, epidemics cannot take off. (This is also why it is possible for selfish free-riders to avoid being vaccinated and still benefit from the fact that most other people have been. )
In The Blind Watchmaker I noted a 'critical mass for explosion' principle at work in human popular culture. Many people choose to buy records, books or clothes for no better reason than that lots of other people are buying them. When a bestseller list is published, this could be seen as an objective report of purchasing behaviour. But it is more than that because the published list feeds back on people's buying behaviour and influences future sales figures. Bestseller lists are therefore, at least potentially, victims of self-feeding spirals. That's why publishers spend
lots of money early in a book's career, in a strenuous attempt to nudge it over the critical threshold of the bestseller list. The hope is that then it will 'take off'. The more you have, the more you get, with the additional feature of sudden take-off, which we need for the purpose of our analogy. A dramatic example of a self-feeding spiral going in the opposite direction is the Wall Street Crash and other cases where panic selling on the stock market feeds on itself in a downward tailspin.
Evolutionary co-adaptation does not necessarily have the additional explosive property of being self-feeding. There is no reason to suppose that, in the evolution of our spider-mimicking fly, the co-adaptation of spider shape and spider behaviour was explosive. In order to be so, it is necessary that the initial resemblance, say a slight anatomical similarity to a spider, set up an increased pressure to mimic the spider's behaviour. This in turn fed an even stronger pressure to mimic the spider's shape, and so on. But, as I say, there is no reason to think it happened like this: no reason to suppose that the pressure was self-feeding and therefore increasing as it shuttled back and forth. As I explained in The Blind Watchmaker, it is possible that the evolution of bird of paradise tails, peacock fans and other extravagant ornaments by sexual selection is genuinely self-feeding and explosive. Here, the principle of 'the more you have, the more you get' may really apply.
In the case of the evolution of the human brain, I suspect that we are looking for something explosive, self-feeding, like the chain reaction of the atomic bomb or the evolution of a bird of paradise tail, rather than like the spider-mimicking fly. The appeal of this idea is its power to explain why, among a set of African ape species with chimpanzee-sized brains, one suddenly raced ahead of the others for no very obvious reason. It is as though a random event nudged the hominid brain over a threshold, something equivalent to a 'critical mass', and then the process took off explosively, because it was self-feeding.
What might this self-feeding process have consisted of? The conjecture I offered in my Royal Institution Christmas Lectures was 'software/hardware co-evolution'. As its name suggests, it can be explained by a computer analogy. Unfortunately for the analogy, Moore's law doesn't seem to be explained by any single self-feeding process. Integrated circuit improvement over the years seems to have been brought about by a messy collection of changes, which makes it puzzling why there is apparently steady exponential improvement. Nevertheless, there surely is some software/hardware co-evolution driving the history of computer advances. In particular, there is something corresponding to bursting through a threshold after a pent-up 'need' has been felt.
In the early days of personal computers they offered only primitive word processing software; mine didn't even 'wrap around' at the end of lines. I was then addicted to machine code programming and (I'm slightly ashamed to admit) went to the lengths of writing my own word processing software, called 'Scrivener', which I used to write The Blind Watchmaker - which would otherwise have been finished sooner! During the development of Scrivener, I became increasingly frustrated by the idea of using the keyboard to move the cursor around the screen. I just wanted to point I toyed with using a joystick, as supplied for computer games, but couldn't work out how to do it. I overwhelmingly felt that the software I wanted to write was held up for want of a critical hardware breakthrough. Later I discovered that the device I desperately needed, but wasn't clever enough to imagine, had in fact been invented much earlier. That device was, of course, the mouse.
The mouse was a hardware advance, conceived in the 1960s by Douglas Engelbart who foresaw that it would make possible a new kind of software. This software innovation we now know, in its developed form, as the Graphical User Interface, or GUI, developed in the 1970s by the brilliantly creative team at Xerox PARC, that Athens of the modern world. It was cultivated into commercial success by Apple in 1985, then copied by other companies under names like VisiOn, GEM and - the most commercially successful today - Windows. The point of the story is that an explosion of ingenious software was, in a sense, pent up, waiting to burst on the world, but it had to wait for a crucial piece of hardware, the mouse. Subsequently, the spread of GUI software placed new demands on hardware, which had to become faster and more capacious to handle the needs of graphics. This in turn allowed a rush of more sophisticated new software, especially software capable of exploiting high-speed graphics. The software/hardware spiral continued and its latest production is the worldwide web. Who knows what may be spawned by future turns of the spiral?
Then if you look forward, it turns out the [computer] power is going to be used for a variety of things. Incremental enhancements and ease of use things, and then occasionally you go over some threshold and something new is possible. That was true with the graphical user interface. Every program, got graphical and every output got graphical, that cost us vast amounts of CPU power and it was worth it. . . In fact, I have my own law of software, Nathan's Law, which is that software grows faster than Moore's Law. And that is why there is a Moore's Law.
NATHAN MYHRVOLD, Chief Technology Officer, Microsoft Corporation (1998)
Returning to the evolution of the human brain, what are we looking for to complete the analogy? A minor improvement in hardware, perhaps a slight increase in brain size, which would have gone unnoticed had it not enabled a new software technique which, in turn, unleashed a blossoming spiral of co-evolution? The new software changed the environment in which brain hardware was subject to natural selection. This gave rise to strong Darwinian pressure to improve and enlarge the hardware, to take advantage of the new software, and a self-feeding spiral was under way, with explosive results.
In the case of the human brain, what might the blossoming advance in software have been? What was the equivalent of the GUI? I'll give the clearest example I can come up with of the kind of thing it might have been, without for a moment committing myself to the view that this was the actual one that inaugurated the spiral. My clear example is language. Nobody knows how it began. There doesn't seem to be anything like syntax in non-human animals and it is hard to imagine evolutionary forerunners of it. Equally obscure is the origin of semantics; of words and their meanings. Sounds that mean things like 'feed me' or 'go away' are commonplace in the animal kingdom, but we humans do something quite different. Like other species, we have a limited repertoire of basic sounds, the phonemes, but we are unique in recombining those sounds, stringing them together in an indefinitely large number of combinations to mean things that are fixed only by arbitrary convention. Human language is open-ended in its semantics: phonemes can be recombined to concoct an indefinitely expanding dictionary of words. And it is open- ended in its syntax, too: words can be recombined in an indefinitely large number of sentences by recursive embedment: 'The man is coming. The man who caught the leopard is coming. The man who caught the leopard which killed the goats is coming. The man who caught the leopard which killed the goats who give us our milk is coming. ' Notice how the sentence grows in the middle while the ends - its fundamentals - stay the same. Each of the embedded subordinate clauses is capable of growing in the same way, and there is no limit to the permissible growth. This kind of potentially infinite enlargement, which is suddenly made possible by a single syntactic innovation, seems to be unique to human language.
Nobody knows whether our ancestors' language went through a prototype stage with a small vocabulary and a simple grammar before gradually evolving to the present point where all the thousands of languages in the world are very complex (some say they are all exactly equally complex, but that sounds too ideologically perfect to be wholly plausible). I am biased towards thinking that it was gradual, but it is not quite obvious that it had to be. Some people think it began suddenly, more or less literally invented by a single genius in a particular place at a particular time. Whether it was gradual or sudden, a similar story of
software/hardware co-evolution could be told. A social world in which there is language is a completely different kind of social world from one in which there is not. The selection pressures on genes will never be the same again. The genes find themselves in a world that is more dramatically different than if an ice age had suddenly struck or some terrible new predator had suddenly arrived in the land. In the new social world where language first burst on the scene, there must have been dramatic natural selection in favour of individuals genetically equipped to exploit the new ways. It is reminiscent of the conclusion of the previous chapter, in which I spoke of genes being selected to survive in the virtual worlds constructed socially by brains. It is almost impossible to overestimate the advantages that could have been enjoyed by individuals able to excel in taking advantage of the new world of language. It is not just that brains became bigger to cope with managing language itself. It is also that the whole world in which our ancestors lived was transformed as a consequence of the invention of speaking.
But I used the example of language just to make the idea of software/hardware co-evolution plausible. It may not have been language that pushed the human brain over its critical threshold for inflation, although I have a hunch that it played an important role. It is controversial whether the sound-modulating hardware in the throat was capable of language at the time when the brain began to swell up. There is some fossil evidence to suggest that our likely ancestors Homo habilis and Homo erectus, because of their relatively undescended larynx, probably were not capable of articulating the full range of vowel sounds that modern throats put at our disposal. Some people take this as indicating that language itself arrived late in our evolution. I think this a rather unimaginative conclusion. If there was software/hardware co- evolution, the brain is not the only hardware that we should expect to have improved in the spiral. The vocal apparatus, too, would have evolved in parallel, and the evolutionary descent of the larynx is one of the hardware changes that language itself would drive. Poor vowels are not the same thing as no vowels at all. Even if Homo erectus speech sounded monotonous by our exacting standards, it could still have served as the arena for the evolution of syntax, semantics and the self- feeding descent of the larynx itself. Homo erectus, incidentally, conceivably made boats as well as fire; we should not underestimate them.
Setting language on one side for a moment, what other software innovations might have nudged our ancestors over the critical threshold and initiated the co-evolutionary escalation? Let me suggest two that could have arisen naturally from our ancestors' evolving fondness for meat and hunting. Agriculture is a recent invention. Most of our hominid ancestors have been hunter gatherers. Those who still subsist from this
ancient way of life are often formidable trackers. They can read patterns of footprints, disturbed vegetation, dung deposits and traces of hair to build up a detailed picture of events over a wide area. A pattern of footprints is a graph, a map, a symbolic representation of a series of incidents in animal behaviour. Remember our hypothetical zoologist, whose ability to reconstruct past environments by reading an animal's body and its DNA justified the statement that an animal is a model of its environment? Mightn't we say something similar of an expert ! Kung San tracker, who has only to read footprints in the Kalahari dirt to reconstruct a detailed pattern, description, or model of animal behaviour in the recent past? Properly read, such spoors amount to maps and pictures, and it seems to me plausible that the ability to read such maps and pictures might have arisen in our ancestors before the origin of speech in words.
Suppose that a band of Homo habilis hunters needed to plan a cooperative hunt. In a remarkable and chilling 1992 television film, Too Close for Comfort, David Attenborough shows modern chimpanzees executing what seems to be a carefully planned and successful drive and ambush of a colobus monkey, which they then tear to pieces and eat. There is no reason to think that the chimpanzees communicated any detailed plan to each other before beginning the hunt, but every reason to think that habilis might have benefited from some such communication if it could have been achieved. How might such communication have developed? Suppose that one of the hunters, whom we can think of as a leader, has a plan to ambush an eland and he wishes to convey the plan to his colleagues. No doubt he could mime the behaviour of the eland, perhaps donning an eland skin for the purpose, as hunting peoples do today for ritual or entertainment purposes. And he could mime the actions he wants his hunters to perform: studied exaggeration of stealth in the stalk; noisy conspicuousness in the drive; sudden startle in the final ambush. But there is more that he could do, and in this he would resemble any modern army officer. He could point out objectives and planning manoeuvres on a map of the area.
Our hunters, we may suppose, are all expert trackers, with a feel for the layout, in two-dimensional space, of footsteps and other traces: a spatial expertise which may have been beyond anything we (unless we happen to be ! Kung San hunters ourselves) can easily imagine. They are all fully accustomed to the idea of following a trail, and imagining it laid out on the ground as a life-size map and a temporal graph of the movements of an animal. What could be more natural than for the leader to seize a stick and draw in the dust a scale model of just such a temporal picture: a map of movement over a surface? The leader and his hunters are fully used to the idea that a series of hoofprints indicate the flow of wildebeests along the muddy bank of a river. Why should he not draw a
line indicating the flow of the river itself on a scale map in the dust? Accustomed as they all are to following human footprints from their own home cave to the river, why would the leader not point on his map to the position of the cave in relation to the river? Moving around the map with his stick, the hunter could indicate the direction of approach by the eland, the angle of his proposed drive, the location of the ambush: indicate them literally by drawing in the sand.
Could something like this have been how the notion of a scaled-down representation in two dimensions was born - as a natural generalization of the important skill of reading animal footprints? Maybe the idea of drawing the likeness of animals themselves arose from the same source. The imprint in mud of a wildebeest hoof is obviously a negative image of the real thing. The fresh paw mark of a lion must have aroused fear. Did it also engender in a blinding flash the realization that one could draw a representation of a part of an animal - and hence, by extrapolation, of the whole animal? Perhaps the blinding flash that led to the first drawing of a whole animal came from the imprint of a whole corpse, dragged out of mud which had baked hard around it. Or a less distinct image in the grass could easily have been fleshed out by the mind's own virtual reality software.
Because the mountain grass Cannot but keep the form Where the mountain hare has lain, W. B. YEATS, 'Memory' (1919)
Representational art of all kinds (and probably non-representational art, too) depends upon noticing that something can be made to stand for something else and that this may assist thought or communication. The analogies and metaphors that underlie what I have been calling poetic science - good and bad - are other manifestations of the same human faculty of symbol-making. Let's recognize a continuum, which could represent an evolutionary series. At one end of the continuum we allow things to stand for other things that they resemble - as in cave paintings of buffaloes. At the other end are symbols which do not obviously resemble the things that they stand for - as in the word 'buffalo', which means what it does only because of an arbitrary convention which all English speakers respect. The intermediate stages along the continuum may, as I said, represent an evolutionary progression. We may never know how it began. But perhaps my story of the footprints represents the kind of insight that might have been involved when people first began to think by analogy, and hence realize the possibility of semantic representation. Whether or not it gave birth to semantics, my tracker map joins language as my second suggestion for a software innovation that may have triggered the co-evolutionary spiral that drove the expansion of our brain. Could it have been the drawing of maps that
boosted our ancestors beyond the critical threshold which the other apes just failed to cross?
My third possible software innovation is inspired by a suggestion made by William Calvin. He proposed that ballistic movements, such as throwing projectiles at a distant target, make special computational demands on nervous tissue. His idea was that the conquering of this particular problem, perhaps originally for purposes of hunting, equipped the brain to do lots of other important things as a by-product.
On a shingle beach, Calvin was amusing himself by tossing - stones at a log and the action inadvertently launched (the metaphor is no accident) a productive train of thought. What kind of computation must the brain be doing when we throw something at a target, as our ancestors must increasingly have done while they evolved the hunting habit? One crucial component of an accurate throw is timing. Whichever arm action you favour, whether underarm lobbing, overarm bowling or throwing, or wristy flicking, the exact moment at which you release your projectile makes all the difference. Think about the overarm action of a bowler in cricket (bowling differs from baseball pitching in that the arm must remain straight, and this makes it easier to think about). If you release the ball too soon, it flies over the batsmen's head. If you let go too late, it digs into the ground. How does the nervous system achieve the feat of releasing the projectile at exactly the right moment, tailored to the speed of arm movement? Unlike a lunge with a sword, in which you might steer your aim all the way to the target, bowling or throwing is ballistic. The projectile leaves your hand and is then beyond your control. There are other skilled movements, like hammering a nail, which are effectively ballistic, even if the tool or weapon doesn't leave your hand. All the computation has to be done in advance: 'dead reckoning'.
One way to solve the release timing problem when throwing a stone or a spear would be to compute the necessary contractions of individual muscles on the fly, while the arm was in motion. Modern digital computers would be capable of this feat, but brains are too slow. Calvin reasoned instead that nervous systems, being slow, would be better off with a buffer store of rote commands to the muscles. The whole sequence of bowling a cricket ball, or throwing a spear, is programmed in the brain as a pre-recorded list of individual muscle twitch commands, packed away in the order they are to be released.
Obviously, more distant targets are harder to hit. Calvin dusted off his physics textbooks and worked out how to calculate the decreasing 'launch window' as you try to maintain accuracy for longer and longer throws. Launch window is space jargon. Rocket scientists (that proverbially gifted profession) calculate the window of opportunity during
which they must launch a spacecraft if they are to hit, say, the moon.
Fire too soon, or too late, and you miss. Calvin worked out that for a rabbit-sized target four metres away, his launch window was about 11 milliseconds wide. If he released his stone too soon, it overshot the rabbit. If he held on too long, his stone fell short. The difference between two short and too long was a mere 11 milliseconds, about a hundredth of a second. Being an expert in the timings of nerve cells, this bothered Calvin, because he knew that the normal margin of error of a nerve cell is greater than the launch window. Yet he also knew that good human throwers are capable of hitting such a target at this distance, even while running. I myself have never forgotten the spectacle of my Oxford contemporary the Nawab of Pataudi (one of India's greatest cricketers, even after losing one eye) fielding for the university and throwing the ball with devastating speed and accuracy at the wicket, again and again, even while running at a speed that visibly intimidated the batsmen while raising the game of his team.
Calvin had a mystery to solve. How do we throw so well? The answer, he decided, must lie in the law of large numbers.
principle underlying these pictures. I won't even try to better his explanation.
There is an easy way to demonstrate that the brain works as a sophisticated virtual reality computer. First, look about you by moving your eyes. As you swivel your eyes, the images on your retinas move as if you were in an earthquake. But you don't see an earthquake. To you, the scene seems as steady as a rock. I am leading up, of course, to saying that the virtual model in your brain is constructed to remain steady. But there is more to the demonstration, because there's another way to make the image on your retina move. Gently poke your eyeball through the skin of the eyelid. The retinal image will move in the same kind of way as before. Indeed you could, given sufficient skill with your finger, mimic the effect of shifting your gaze. But now you really will think you see the earth move. The whole scene shifts, as if you were witnessing an earthquake.
What is the difference between these two cases? It is that the brain computer has been set up to take account of normal eye movements and make allowance for them in constructing its computed model of the world. Apparently the brain model makes use of information, not only from the eyes, but also from the instructions to move the eyes. Whenever the brain issues an order to the eye muscles to move the eye, a copy of that order is sent to the part of the brain that is constructing the internal model of the world. Then, when the eyes move, the virtual reality software of the brain is warned to expect the retinal images to move just the right amount, and it makes the model compensate. So the constructed model of the world is seen to stay still, although it may be viewed from another angle. If the earth moves at any time other than when the model is told to expect movement, the virtual model moves accordingly. This is fine, because there really might be an earthquake. Except that you can fool the system by poking your eyeball. As the final demonstration using yourself as guinea pig, make yourself giddy by spinning round and round. Now stand still and look fixedly at the world. It will appear to spin even though your reason tells you that it is not getting anywhere in its rotation. Your retinal images are not moving, but the accelerometers in your ears (which work by detecting the movements of fluid in the so-called semicircular canals) are telling the brain that you are spinning. The brain instructs the virtual reality software to expect to see the world spinning. When the images on the retina do not spin, therefore, the model registers the discrepancy and spins itself in the opposite direction. To put it in subjective language, the virtual reality software says to itself, 'I know I'm spinning from what the ears are telling me; therefore, in order to hold the model still, it will be necessary to put the opposite spin on the model, relative to the data that the eyes are sending in. ' But the retinas actually report no spin, so the compensating
spin of the model in the head is what you seem to see. In Barlow's terms, it is the unexpected, it is 'news', and that is why we see it.
Birds have an additional problem which humans ordinarily are spared. A bird perched on a tree branch is constantly being blown up and down, to and fro, and its retinal images seesaw accordingly. It is like living through a permanent earthquake. Birds keep their heads, and hence their view of the world, steady by diligent use of the neck muscles. If you film a bird on a windblown branch, you can almost imagine that the head is nailed to the background, while the neck muscles use the head as a fulcrum to move the rest of the body. When a bird walks, it employs the same trick to keep its perceived world steady. That is why walking chickens jerk their heads back and forth in what can seem to us quite a comical fashion. It is actually rather clever. As the body moves forward, the neck draws the head backwards in a controlled way so that the retinal images remain steady. Then the head shoots forward to allow the cycle to repeat. I can't help wondering whether, as an untoward consequence of the bird way of doing things, a bird might be unable to see a real earthquake because its neck muscles would automatically compensate. More seriously, we might say that the bird is using its neck muscles in a Barlow-style exercise: holding the non-newsworthy part of the world constant so that genuine movement stands out.
Insects and many other animals seem to have a similar habit of working to keep their visual world constant. Experimenters have demonstrated this in a so-called 'optomotor apparatus', where the insect is placed on a table and surrounded by a hollow cylinder painted on the inside with vertical stripes. If you now rotate the cylinder, the insect will use its legs to turn, keeping up with the cylinder. It is working to keep its visual world constant.
Normally, an insect has to tell its simulating software to expect movement when it walks, otherwise it would start compensating for its own movements, and then where would it be? This thought prompted two ingenious Germans, Erich von Hoist and Horst Mittelstaedt, to a diabolically cunning experiment. If you've ever watched a fly washing its face with its hands, you will know that flies are capable of flicking their head completely upside down. Von Hoist and Mittelstaedt succeeded in fixing a fly's head in the inverted position using glue. You have already guessed the consequence. Normally, whenever a fly turns its body, the model in its brain is told to expect a corresponding movement of the visual world. But as soon as it took a step, the wretched fly with its head upside down received data suggesting that the world had moved in the opposite direction to the one expected. It therefore moved its legs further in the same direction in order to compensate. This caused the apparent position of the world to move even further. The fly ended up spinning
round and round like a top, at ever-increasing speed - well, within obvious practical limits.
The same Erich von Hoist also pointed out that we should expect a similar confusion if our own voluntary instructions to move our eyes are neutralized, for example by narcotizing the eye-moving muscles. Normally, if you give your eyes the command to move to the right, your retinal images will signal a move to the left. To compensate and create the appearance of stability, the model in the head has to be moved to the right. But if the eye-moving muscles are narcotized, the model should move to the right in anticipation of what turns out to be a non-existent retinal movement. Let von Hoist himself take up the story, in his paper 'The Behavioural Physiology of Animals and Man' (1973):
This is indeed the case! It has been known for many years from people with paralysed eye muscles and it has been established exactly from the experiments of Kornmuller on himself that every intended but unfulfilled eye movement results in the perception of a quantitative movement of the surroundings in the same direction.
We are so used to living in our simulated world and it is kept so beautifully in synchrony with the real world that we don't realize it is a simulated world. It takes clever experiments like those of von Hoist and his colleagues to bring it home to us.
And it has its dark side. A brain that is good at simulating models in imagination is also, almost inevitably, in danger of self-delusion. How many of us as children have lain in bed, terrified because we thought we saw a ghost or a monstrous face staring in at the bedroom window, only to discover that it was a trick of the light? I've already discussed how eagerly our brain's simulation software will construct a solid face where the reality is a hollow face. It will just as eagerly make a ghostly face where the reality is a collection of moonlit folds in a white net curtain.
Every night of our lives we dream. Our simulation software sets up
worlds that do not exist; people, animals and places that never existed, perhaps never could exist. At the time, we experience these simulations as though they were reality. Why should we not, given that we habitually experience reality in the same way - as simulation models? The simulation software can delude us when we are awake, too. Illusions like the hollow face are in themselves harmless, and we understand how they work. But our simulation software can also, if we are drugged, or feverish, or fasting, produce hallucinations. Throughout history, people have seen visions of angels, saints and gods; and these have seemed very real to them. Well, of course they would seem real. They are models, put
together by the normal simulation software. The simulation software is
using the same modelling techniques as it uses ordinarily when it presents its continuously updated edition of reality. No wonder these visions have been so influential. No wonder they have changed people's lives. So if ever we hear a story that somebody has seen a vision, been visited by an archangel, or heard voices in the head, we should immediately be suspicious of taking it at face value. Remember that all our heads contain powerful and ultra-realistic simulation software. Our simulation software could knock up a ghost or a dragon or a saintly virgin in no time flat. It would be child's play for software of that sophistication.
A word of warning. The metaphor of virtual reality is beguiling and, in many ways, apt. But there is a danger of its misleading us into thinking that there is a 'little man' or 'homunculus' in the brain watching the virtual reality show. As philosophers such as Daniel Dennett have pointed out, you have explained precisely nothing if you suggest that the eye is wired to the brain in such a way that a little cinema screen, somewhere in the brain, continuously relays whatever is projected on the retina. Who looks at the screen? The question now raised is no smaller than the original question you think you have answered. You might as well let the little man look at the retina directly, which is clearly no solution to anything. The same problem arises if we take the virtual reality metaphor literally and imagine that some agent locked inside the head is 'experiencing' the virtual reality performance.
The problems raised by subjective consciousness are perhaps the most baffling in all philosophy, and solving them is far beyond my ambition. My suggestion is the more modest one that each species, in each situation, needs to deploy its information about the world in whatever way is most useful for taking action. 'Constructing a model in the head' is a helpful way to express how it is done, and comparing it to virtual reality is especially helpful in the case of humans. As I have argued before, the model of the world used by a bat is likely to be similar to the model used by a swallow, even though one is connected to the real world via the ears, the other via the eyes. The brain constructs its model world in the way most suited for action. Since the actions of day-flying swallows and night-flying bats are similar - navigating at high speed in three dimensions, avoiding solid obstacles and catching insects on the wing - they are likely to use the same models. I do not postulate a 'little bat in the head' or a 'little swallow in the head' to watch the model. Somehow the model is used to control the wing muscles, and that is as far as I go.
Nevertheless, each of us humans knows that the illusion of a single agent sitting somewhere in the middle of the brain is a powerful one. I suspect that the case may be parallel to the 'selfish Cooperator' model of
genes coming together, although they are fundamentally independent agents, to create the illusion of a unitary body. I'll briefly return to the idea near the end of the next chapter.
This chapter has developed the thesis that brains have taken over from DNA part of the role of recording the environment - environments, rather, for they are many and spread out over the near and the distant past. Having a record of the past is useful only in so far as it helps in predicting the future. The animal's body represents a kind of prediction that the future will resemble the ancestral past, in broad outline. The animal is likely to survive to the extent that this turns out to be true. And simulation models of the world allow the animal to act as if in anticipation of what that world is likely to throw its way in the next few seconds, hours or days. For completeness we must note that the brain itself, and its virtual reality software, are ultimately the products of natural selection of ancestral genes. We could say that the genes can predict a limited amount, because only in a general way will the future resemble the past. For the details and the subtleties, they provide the animal with nervous hardware and virtual reality software which will constantly update and revise its predictions to fit highspeed changes in circumstances. It is as if the genes say, 'We can model the basic shape of the environment, the things that don't change over the generations. But for the fast changes, over to you, brain. '
We move through a virtual world of our own brains' making. Our constructed models of rocks and of trees are a part of the environment in which we animals live, no less than the real rocks and trees that they represent. And, intriguingly, our virtual worlds must also be seen as part of the environment in which our genes are naturally selected. We have pictured camel genes as denizens of ancestral worlds, selected to survive in ancient deserts and even more ancient seas, selected to survive in companionship with compatible cartels of other camel genes. All that is true, and equivalent stories of Miocene trees and Pliocene savannahs can be told of our genes. What we must now add is that, among the worlds in which genes have survived are virtual worlds constructed inside ancestral brains.
In the case of highly social animals like ourselves and our ancestors, our virtual worlds are, at least in part, group constructions. Especially since the invention of language and the rise of artifact and technology, our genes have had to survive in complex and changing worlds for which the most economical description we can find is shared virtual reality. It is a startling thought that, just as genes can be said to survive in desserts or forests, and just as they can be said to survive in the company of other genes in the gene pool, genes can also be said to survive in the virtual,
even poetic worlds created by brains. It is to the enigma of the human brain itself that we turn in the final chapter.
12
THE BALLOON OF THE MIND
The brain is a three pound mass you can hold in your hand that can conceive of a universe a hundred billion light-years across.
MARIAN C. DIAMOND
It is a commonplace among historians of science that the biologists of any age, struggling to understand the workings of living bodies, make comparison with the advanced technology of their time. From clocks in the seventeenth century to dancing statues in the eighteenth, from Victorian heat engines to today's heat-seeking, electronically guided missiles, the engineering novelties of every age have refreshed the biological imagination. If, of all these innovations, the digital computer promises to overshadow its predecessors, the reason is simple. The computer is not just one machine. It can be swiftly reprogrammed to become any machine you like: calculator, word processor, card index, chess master, musical instrument, guess-your-weight machine, even, I regret to say, astrological soothsayer. It can simulate the weather, lemming population cycles, an ants' nest, satellite docking, or the city of Vancouver.
The brain of any animal has been described as its on-board computer. It does not work in the same way as an electronic computer. It is made
from very different components. These are individually much slower, but they work in huge parallel networks so that, by some means still only partly understood, their numbers compensate for their slower speed, and brains can, in certain respects, outperform digital computers. In any case, the differences of detailed working do not disempower the metaphor. The brain is the body's on-board computer, not because of how it works but because of what it does in the life of the animal. The resemblance of role extends to many parts of the animal's economy but, perhaps most spectacularly of all, the brain simulates the world with the equivalent of virtual reality software.
It might seem a good idea, in a general way, for any animal to grow a large brain. Isn't greater computing power always likely to be an advantage? Maybe, but it has costs, too. Weight for weight, brain tissue consumes more energy than other tissues. And our big brains as babies make it quite difficult for us to be born. Our presumption that braininess must be a good thing partly grows out of vanity in our species' own
hypertrophy of the brain. But it remains an interesting question why human brains have grown so especially big.
One authority has said that the evolution of the human brain over the last million years or so is 'perhaps tile fastest advance recorded for any complex organ in the entire history of life'. This may be an exaggeration, but the evolution of the human brain is undeniably fast. Compared with the skulls of other apes, the modern human skull, at least the bulbous part that houses the brain, has blown up like a balloon. When we ask why this happened, it is not satisfactory to produce general reasons why having a large brain might be useful. Presumably such general benefits would apply to many kinds of animal, especially those that navigate rapidly through the complicated three-dimensional world of the forest canopy, as most primates do. A satisfying explanation will be one that tells us why one particular lineage of apes - actually, one that had left the trees - suddenly took off, leaving the rest of the primates standing. It was once fashionable to lament - or, according to taste, gloat over - the paucity of fossils linking Homo sapiens to our ape ancestors. This has changed. We now have a rather good fossil series and as we go backwards in time we can trace a gradual shrinkage in braincase through various species of Homo to our predecessor genus Australopithecus whose braincase was about the same size as a modern chimpanzee's. The main difference between Lucy or Mrs Pies (famous Australopithecines) and a chimpanzee lay not in the brain at all, but in the Australopithecine habit of walking upright on two legs. Chimps only occasionally do. The blowing up of the brain balloon spanned three million years from Australopithecus through Homo habilis, then Homo erectus, through archaic Homo sapiens to modern Homo sapiens.
Something a bit similar seems to have happened in the growth of the computer. But, if the human brain has blown up like a balloon, the computer's progress has been more like an atom bomb. Moore's law states that the capacity of computers of a given physical size doubles every 1. 5 years. (This is a modern version of the law. When Moore originally stated it more than three decades ago he was referring to transistor counts which, on his measurements, doubled every two years. Computer performance has improved even faster because transistors became faster as well as smaller and cheaper. ) The late Christopher Evans, a computer-literate psychologist, put the point dramatically:
Today's car differs from those of the immediate post-war years on a number of counts. It is cheaper, allowing for the ravages of inflation, and it is more economical and efficient. . . But suppose for a moment that the automobile industry had developed at the same rate as computers and over the same period: how much cheaper and more efficient would the current models be? If you have not already heard the analogy the answer
is shattering. Today you would be able to buy a Rolls-Royce for ? 1. 35, it would do three million miles to the gallon, and it would deliver enough power to drive the Queen Elizabeth II. And if you were interested in miniaturization, you could place half a dozen of them on a pinhead. The Mighty Micro (1979)
Of course, things on the timescale of biological evolution inevitably happen far more slowly. One reason is that every improvement has to come about through individuals dying and rival individuals reproducing. So comparisons of absolute speed cannot be made. If we compare the brains of Australopithecus, Homo habilis, Homo erectus and Homo sapiens, we get a rough equivalent of Moore's law, slowed down by six orders of magnitude. From Lucy to Homo sapiens, brain size has approximately doubled every 1. 5 million years. Unlike Moore's law for computers, there is no particular reason to think that the human brain will go on swelling. In order for this to happen, large-brained individuals have to have more children than small-brained individuals. It isn't obvious that this is now happening. It must have happened during our ancestral past, otherwise our brains would not have grown as they did. It also must have been true, incidentally, that braininess in our ancestors was under genetic control. If it had not been, natural selection would have had nothing to work on, and the evolutionary growth of the brain would not have occurred. For some reason, many people take grave political offence at the suggestion that some individuals are genetically cleverer than others. But this must have been the case when our brains were evolving, and there is no reason to expect that facts will suddenly change to accommodate political sensitivities.
Lots of influences have contributed to computer development which are not going to help us to understand brains. A major step was the change from the valve (vacuum tube) to the much smaller transistor, and then the spectacular and continuing miniaturization of the transistor in integrated circuits. These advances are all irrelevant to brains, because - the point deserves repetition - brains don't work electronically anyway. But there is another source of computer advancement, and this might be relevant to brains. I'll call it self-feeding co-evolution.
We have already met co-evolution. It means the evolving together of different organisms (as in the arms races between predators and prey), or between different parts of the same organism (the special case called co- adaptation). As another example, there are some small flies whose appearance mimics that of a jumping spider, including large dummy eyes looking straight forward like paired headlights - very unlike the compound eyes with which the flies themselves see. Real spiders are potential predators of flies of this size, but they are put off by the flies' similarity to another spider. The flies enhance the mimicry by waving
their arms in ways that resemble the histrionic semaphore signals that jumping spiders use when courting their own opposite sex. In the fly, genes controlling the anatomical resemblance to spiders must have evolved together with separate genes controlling the semaphoring behaviour. This evolving together is co-adaptation.
Self-feeding is the name I am giving to any process in which 'the more
you have, the more you get'. A bomb is a good example. The atomic bomb is said to depend upon a chain reaction, but the metaphor of a chain is too stately to convey what happens. When the unstable nucleus of uranium 255 breaks up, energy is released. Neutrons shooting out from the break-up of one nucleus may hit another and induce it to break up
as well, but that is usually the end of the story. Most of the neutrons
miss other nuclei and shoot off harmlessly into empty space, for uranium, though one of the densest of metals, is 'really', like all matter, mostly empty space. (The virtual model of metal in our brains is constructed
with the persuasive illusion of dense solidity because that is the most useful internal representation of a solid for our survival purposes. ) On their own scale, the atomic nuclei in a metal are far more spaced out
than gnats in a swarm, and a particle expelled by one decaying atom is quite likely to have a clear run out of the swarm. If, however, you pack in a quantity (the famous 'critical mass') of uranium 235 which is just sufficient to see to it that a typical neutron expelled from any one
nucleus is on average likely to hit one other nucleus before leaving the mass of metal altogether, a so-called chain reaction gets going. On average, each nucleus that splits causes another to split, there is an epidemic of atom-splitting, with an exceedingly rapid release of heat and other destructive energy, and the results are only too well known. All explosions have this same epidemic quality find, on a slower time-scale, epidemics of disease sometimes resemble explosions. They require a critical mass of susceptible victims in order to get started and, once they do get started, the more you have the more you get. This is why it is so important to vaccinate a critical proportion of the population. If fewer than the 'critical mass' remain unvaccinated, epidemics cannot take off. (This is also why it is possible for selfish free-riders to avoid being vaccinated and still benefit from the fact that most other people have been. )
In The Blind Watchmaker I noted a 'critical mass for explosion' principle at work in human popular culture. Many people choose to buy records, books or clothes for no better reason than that lots of other people are buying them. When a bestseller list is published, this could be seen as an objective report of purchasing behaviour. But it is more than that because the published list feeds back on people's buying behaviour and influences future sales figures. Bestseller lists are therefore, at least potentially, victims of self-feeding spirals. That's why publishers spend
lots of money early in a book's career, in a strenuous attempt to nudge it over the critical threshold of the bestseller list. The hope is that then it will 'take off'. The more you have, the more you get, with the additional feature of sudden take-off, which we need for the purpose of our analogy. A dramatic example of a self-feeding spiral going in the opposite direction is the Wall Street Crash and other cases where panic selling on the stock market feeds on itself in a downward tailspin.
Evolutionary co-adaptation does not necessarily have the additional explosive property of being self-feeding. There is no reason to suppose that, in the evolution of our spider-mimicking fly, the co-adaptation of spider shape and spider behaviour was explosive. In order to be so, it is necessary that the initial resemblance, say a slight anatomical similarity to a spider, set up an increased pressure to mimic the spider's behaviour. This in turn fed an even stronger pressure to mimic the spider's shape, and so on. But, as I say, there is no reason to think it happened like this: no reason to suppose that the pressure was self-feeding and therefore increasing as it shuttled back and forth. As I explained in The Blind Watchmaker, it is possible that the evolution of bird of paradise tails, peacock fans and other extravagant ornaments by sexual selection is genuinely self-feeding and explosive. Here, the principle of 'the more you have, the more you get' may really apply.
In the case of the evolution of the human brain, I suspect that we are looking for something explosive, self-feeding, like the chain reaction of the atomic bomb or the evolution of a bird of paradise tail, rather than like the spider-mimicking fly. The appeal of this idea is its power to explain why, among a set of African ape species with chimpanzee-sized brains, one suddenly raced ahead of the others for no very obvious reason. It is as though a random event nudged the hominid brain over a threshold, something equivalent to a 'critical mass', and then the process took off explosively, because it was self-feeding.
What might this self-feeding process have consisted of? The conjecture I offered in my Royal Institution Christmas Lectures was 'software/hardware co-evolution'. As its name suggests, it can be explained by a computer analogy. Unfortunately for the analogy, Moore's law doesn't seem to be explained by any single self-feeding process. Integrated circuit improvement over the years seems to have been brought about by a messy collection of changes, which makes it puzzling why there is apparently steady exponential improvement. Nevertheless, there surely is some software/hardware co-evolution driving the history of computer advances. In particular, there is something corresponding to bursting through a threshold after a pent-up 'need' has been felt.
In the early days of personal computers they offered only primitive word processing software; mine didn't even 'wrap around' at the end of lines. I was then addicted to machine code programming and (I'm slightly ashamed to admit) went to the lengths of writing my own word processing software, called 'Scrivener', which I used to write The Blind Watchmaker - which would otherwise have been finished sooner! During the development of Scrivener, I became increasingly frustrated by the idea of using the keyboard to move the cursor around the screen. I just wanted to point I toyed with using a joystick, as supplied for computer games, but couldn't work out how to do it. I overwhelmingly felt that the software I wanted to write was held up for want of a critical hardware breakthrough. Later I discovered that the device I desperately needed, but wasn't clever enough to imagine, had in fact been invented much earlier. That device was, of course, the mouse.
The mouse was a hardware advance, conceived in the 1960s by Douglas Engelbart who foresaw that it would make possible a new kind of software. This software innovation we now know, in its developed form, as the Graphical User Interface, or GUI, developed in the 1970s by the brilliantly creative team at Xerox PARC, that Athens of the modern world. It was cultivated into commercial success by Apple in 1985, then copied by other companies under names like VisiOn, GEM and - the most commercially successful today - Windows. The point of the story is that an explosion of ingenious software was, in a sense, pent up, waiting to burst on the world, but it had to wait for a crucial piece of hardware, the mouse. Subsequently, the spread of GUI software placed new demands on hardware, which had to become faster and more capacious to handle the needs of graphics. This in turn allowed a rush of more sophisticated new software, especially software capable of exploiting high-speed graphics. The software/hardware spiral continued and its latest production is the worldwide web. Who knows what may be spawned by future turns of the spiral?
Then if you look forward, it turns out the [computer] power is going to be used for a variety of things. Incremental enhancements and ease of use things, and then occasionally you go over some threshold and something new is possible. That was true with the graphical user interface. Every program, got graphical and every output got graphical, that cost us vast amounts of CPU power and it was worth it. . . In fact, I have my own law of software, Nathan's Law, which is that software grows faster than Moore's Law. And that is why there is a Moore's Law.
NATHAN MYHRVOLD, Chief Technology Officer, Microsoft Corporation (1998)
Returning to the evolution of the human brain, what are we looking for to complete the analogy? A minor improvement in hardware, perhaps a slight increase in brain size, which would have gone unnoticed had it not enabled a new software technique which, in turn, unleashed a blossoming spiral of co-evolution? The new software changed the environment in which brain hardware was subject to natural selection. This gave rise to strong Darwinian pressure to improve and enlarge the hardware, to take advantage of the new software, and a self-feeding spiral was under way, with explosive results.
In the case of the human brain, what might the blossoming advance in software have been? What was the equivalent of the GUI? I'll give the clearest example I can come up with of the kind of thing it might have been, without for a moment committing myself to the view that this was the actual one that inaugurated the spiral. My clear example is language. Nobody knows how it began. There doesn't seem to be anything like syntax in non-human animals and it is hard to imagine evolutionary forerunners of it. Equally obscure is the origin of semantics; of words and their meanings. Sounds that mean things like 'feed me' or 'go away' are commonplace in the animal kingdom, but we humans do something quite different. Like other species, we have a limited repertoire of basic sounds, the phonemes, but we are unique in recombining those sounds, stringing them together in an indefinitely large number of combinations to mean things that are fixed only by arbitrary convention. Human language is open-ended in its semantics: phonemes can be recombined to concoct an indefinitely expanding dictionary of words. And it is open- ended in its syntax, too: words can be recombined in an indefinitely large number of sentences by recursive embedment: 'The man is coming. The man who caught the leopard is coming. The man who caught the leopard which killed the goats is coming. The man who caught the leopard which killed the goats who give us our milk is coming. ' Notice how the sentence grows in the middle while the ends - its fundamentals - stay the same. Each of the embedded subordinate clauses is capable of growing in the same way, and there is no limit to the permissible growth. This kind of potentially infinite enlargement, which is suddenly made possible by a single syntactic innovation, seems to be unique to human language.
Nobody knows whether our ancestors' language went through a prototype stage with a small vocabulary and a simple grammar before gradually evolving to the present point where all the thousands of languages in the world are very complex (some say they are all exactly equally complex, but that sounds too ideologically perfect to be wholly plausible). I am biased towards thinking that it was gradual, but it is not quite obvious that it had to be. Some people think it began suddenly, more or less literally invented by a single genius in a particular place at a particular time. Whether it was gradual or sudden, a similar story of
software/hardware co-evolution could be told. A social world in which there is language is a completely different kind of social world from one in which there is not. The selection pressures on genes will never be the same again. The genes find themselves in a world that is more dramatically different than if an ice age had suddenly struck or some terrible new predator had suddenly arrived in the land. In the new social world where language first burst on the scene, there must have been dramatic natural selection in favour of individuals genetically equipped to exploit the new ways. It is reminiscent of the conclusion of the previous chapter, in which I spoke of genes being selected to survive in the virtual worlds constructed socially by brains. It is almost impossible to overestimate the advantages that could have been enjoyed by individuals able to excel in taking advantage of the new world of language. It is not just that brains became bigger to cope with managing language itself. It is also that the whole world in which our ancestors lived was transformed as a consequence of the invention of speaking.
But I used the example of language just to make the idea of software/hardware co-evolution plausible. It may not have been language that pushed the human brain over its critical threshold for inflation, although I have a hunch that it played an important role. It is controversial whether the sound-modulating hardware in the throat was capable of language at the time when the brain began to swell up. There is some fossil evidence to suggest that our likely ancestors Homo habilis and Homo erectus, because of their relatively undescended larynx, probably were not capable of articulating the full range of vowel sounds that modern throats put at our disposal. Some people take this as indicating that language itself arrived late in our evolution. I think this a rather unimaginative conclusion. If there was software/hardware co- evolution, the brain is not the only hardware that we should expect to have improved in the spiral. The vocal apparatus, too, would have evolved in parallel, and the evolutionary descent of the larynx is one of the hardware changes that language itself would drive. Poor vowels are not the same thing as no vowels at all. Even if Homo erectus speech sounded monotonous by our exacting standards, it could still have served as the arena for the evolution of syntax, semantics and the self- feeding descent of the larynx itself. Homo erectus, incidentally, conceivably made boats as well as fire; we should not underestimate them.
Setting language on one side for a moment, what other software innovations might have nudged our ancestors over the critical threshold and initiated the co-evolutionary escalation? Let me suggest two that could have arisen naturally from our ancestors' evolving fondness for meat and hunting. Agriculture is a recent invention. Most of our hominid ancestors have been hunter gatherers. Those who still subsist from this
ancient way of life are often formidable trackers. They can read patterns of footprints, disturbed vegetation, dung deposits and traces of hair to build up a detailed picture of events over a wide area. A pattern of footprints is a graph, a map, a symbolic representation of a series of incidents in animal behaviour. Remember our hypothetical zoologist, whose ability to reconstruct past environments by reading an animal's body and its DNA justified the statement that an animal is a model of its environment? Mightn't we say something similar of an expert ! Kung San tracker, who has only to read footprints in the Kalahari dirt to reconstruct a detailed pattern, description, or model of animal behaviour in the recent past? Properly read, such spoors amount to maps and pictures, and it seems to me plausible that the ability to read such maps and pictures might have arisen in our ancestors before the origin of speech in words.
Suppose that a band of Homo habilis hunters needed to plan a cooperative hunt. In a remarkable and chilling 1992 television film, Too Close for Comfort, David Attenborough shows modern chimpanzees executing what seems to be a carefully planned and successful drive and ambush of a colobus monkey, which they then tear to pieces and eat. There is no reason to think that the chimpanzees communicated any detailed plan to each other before beginning the hunt, but every reason to think that habilis might have benefited from some such communication if it could have been achieved. How might such communication have developed? Suppose that one of the hunters, whom we can think of as a leader, has a plan to ambush an eland and he wishes to convey the plan to his colleagues. No doubt he could mime the behaviour of the eland, perhaps donning an eland skin for the purpose, as hunting peoples do today for ritual or entertainment purposes. And he could mime the actions he wants his hunters to perform: studied exaggeration of stealth in the stalk; noisy conspicuousness in the drive; sudden startle in the final ambush. But there is more that he could do, and in this he would resemble any modern army officer. He could point out objectives and planning manoeuvres on a map of the area.
Our hunters, we may suppose, are all expert trackers, with a feel for the layout, in two-dimensional space, of footsteps and other traces: a spatial expertise which may have been beyond anything we (unless we happen to be ! Kung San hunters ourselves) can easily imagine. They are all fully accustomed to the idea of following a trail, and imagining it laid out on the ground as a life-size map and a temporal graph of the movements of an animal. What could be more natural than for the leader to seize a stick and draw in the dust a scale model of just such a temporal picture: a map of movement over a surface? The leader and his hunters are fully used to the idea that a series of hoofprints indicate the flow of wildebeests along the muddy bank of a river. Why should he not draw a
line indicating the flow of the river itself on a scale map in the dust? Accustomed as they all are to following human footprints from their own home cave to the river, why would the leader not point on his map to the position of the cave in relation to the river? Moving around the map with his stick, the hunter could indicate the direction of approach by the eland, the angle of his proposed drive, the location of the ambush: indicate them literally by drawing in the sand.
Could something like this have been how the notion of a scaled-down representation in two dimensions was born - as a natural generalization of the important skill of reading animal footprints? Maybe the idea of drawing the likeness of animals themselves arose from the same source. The imprint in mud of a wildebeest hoof is obviously a negative image of the real thing. The fresh paw mark of a lion must have aroused fear. Did it also engender in a blinding flash the realization that one could draw a representation of a part of an animal - and hence, by extrapolation, of the whole animal? Perhaps the blinding flash that led to the first drawing of a whole animal came from the imprint of a whole corpse, dragged out of mud which had baked hard around it. Or a less distinct image in the grass could easily have been fleshed out by the mind's own virtual reality software.
Because the mountain grass Cannot but keep the form Where the mountain hare has lain, W. B. YEATS, 'Memory' (1919)
Representational art of all kinds (and probably non-representational art, too) depends upon noticing that something can be made to stand for something else and that this may assist thought or communication. The analogies and metaphors that underlie what I have been calling poetic science - good and bad - are other manifestations of the same human faculty of symbol-making. Let's recognize a continuum, which could represent an evolutionary series. At one end of the continuum we allow things to stand for other things that they resemble - as in cave paintings of buffaloes. At the other end are symbols which do not obviously resemble the things that they stand for - as in the word 'buffalo', which means what it does only because of an arbitrary convention which all English speakers respect. The intermediate stages along the continuum may, as I said, represent an evolutionary progression. We may never know how it began. But perhaps my story of the footprints represents the kind of insight that might have been involved when people first began to think by analogy, and hence realize the possibility of semantic representation. Whether or not it gave birth to semantics, my tracker map joins language as my second suggestion for a software innovation that may have triggered the co-evolutionary spiral that drove the expansion of our brain. Could it have been the drawing of maps that
boosted our ancestors beyond the critical threshold which the other apes just failed to cross?
My third possible software innovation is inspired by a suggestion made by William Calvin. He proposed that ballistic movements, such as throwing projectiles at a distant target, make special computational demands on nervous tissue. His idea was that the conquering of this particular problem, perhaps originally for purposes of hunting, equipped the brain to do lots of other important things as a by-product.
On a shingle beach, Calvin was amusing himself by tossing - stones at a log and the action inadvertently launched (the metaphor is no accident) a productive train of thought. What kind of computation must the brain be doing when we throw something at a target, as our ancestors must increasingly have done while they evolved the hunting habit? One crucial component of an accurate throw is timing. Whichever arm action you favour, whether underarm lobbing, overarm bowling or throwing, or wristy flicking, the exact moment at which you release your projectile makes all the difference. Think about the overarm action of a bowler in cricket (bowling differs from baseball pitching in that the arm must remain straight, and this makes it easier to think about). If you release the ball too soon, it flies over the batsmen's head. If you let go too late, it digs into the ground. How does the nervous system achieve the feat of releasing the projectile at exactly the right moment, tailored to the speed of arm movement? Unlike a lunge with a sword, in which you might steer your aim all the way to the target, bowling or throwing is ballistic. The projectile leaves your hand and is then beyond your control. There are other skilled movements, like hammering a nail, which are effectively ballistic, even if the tool or weapon doesn't leave your hand. All the computation has to be done in advance: 'dead reckoning'.
One way to solve the release timing problem when throwing a stone or a spear would be to compute the necessary contractions of individual muscles on the fly, while the arm was in motion. Modern digital computers would be capable of this feat, but brains are too slow. Calvin reasoned instead that nervous systems, being slow, would be better off with a buffer store of rote commands to the muscles. The whole sequence of bowling a cricket ball, or throwing a spear, is programmed in the brain as a pre-recorded list of individual muscle twitch commands, packed away in the order they are to be released.
Obviously, more distant targets are harder to hit. Calvin dusted off his physics textbooks and worked out how to calculate the decreasing 'launch window' as you try to maintain accuracy for longer and longer throws. Launch window is space jargon. Rocket scientists (that proverbially gifted profession) calculate the window of opportunity during
which they must launch a spacecraft if they are to hit, say, the moon.
Fire too soon, or too late, and you miss. Calvin worked out that for a rabbit-sized target four metres away, his launch window was about 11 milliseconds wide. If he released his stone too soon, it overshot the rabbit. If he held on too long, his stone fell short. The difference between two short and too long was a mere 11 milliseconds, about a hundredth of a second. Being an expert in the timings of nerve cells, this bothered Calvin, because he knew that the normal margin of error of a nerve cell is greater than the launch window. Yet he also knew that good human throwers are capable of hitting such a target at this distance, even while running. I myself have never forgotten the spectacle of my Oxford contemporary the Nawab of Pataudi (one of India's greatest cricketers, even after losing one eye) fielding for the university and throwing the ball with devastating speed and accuracy at the wicket, again and again, even while running at a speed that visibly intimidated the batsmen while raising the game of his team.
Calvin had a mystery to solve. How do we throw so well? The answer, he decided, must lie in the law of large numbers.
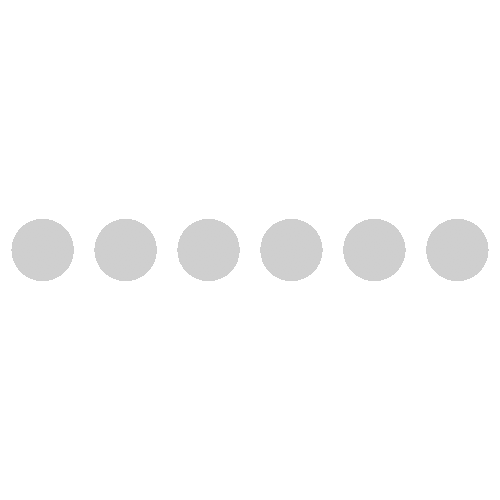