She rightly grants
bacteria
centre stage among life forms on our planet.
Richard-Dawkins-Unweaving-the-Rainbow
Species A genes that work well against a genetic background or 'climate' of other Species A genes do not work when transplanted into Species B, and vice versa.
Similar effects are sometimes seen when varieties or races within one species hybridize.
I first understood this while listening to lectures by the late E. B. Ford, legendary Oxford aesthete and eccentric founder of the now neglected school of Ecological Geneticists. Most of Ford's research was on wild populations of butterflies and moths. Among these was the Lesser Yellow Underwing moth, Triphaena comes. This moth is normally yellowish brown, but there is a variant called curtisii which is blackish. Curtisii is not found in England at all; however, in Scotland and the Isles curtisii coexists with the normal comes. The curtisii dark colour pattern is nearly completely dominant to the normal comes pattern. 'Dominant to' is a technical term, which is why I can't just say 'dominates'. It means that hybrids between the two look like curtisii even though they bear the genes of both. Ford caught specimens from Barra in the Outer Hebrides, west of Scotland, and from one of the Orkney islands, north of Scotland, as well as from the Scottish mainland itself. Each of the two island forms looks exactly like its opposite number at the other island site, and the dark curtisii gene is dominant on the two islands, as well as on the mainland. Other evidence shows that the curtisii gene is the very same gene in all localities. In view of this you'd expect that, when you cross- bred specimens from different islands, the normal dominance pattern would hold up. But it doesn't, and this is the point of the story. Ford caught individuals from Barra and mated them with individuals from Orkney. And the dominance of curtisii completely disappeared. A full range of intermediates turned up in the hybrid families, just as if there was no dominance. What seems to be going on is this. The curtisii gene does not, in itself, encode the formula for the coloured pigment by which we distinguish the moths, nor is dominance ever a property of a gene on its own. Instead, like any other gene, the curtisii gene should be thought
of as having its effects only in the context of a suite of other genes, some of which it 'switches on'. This suite of other genes is part of what I mean by 'genetic background' or 'genetic climate'. In theory, any gene could therefore exert radically different effects on different islands, in the presence of different suites of other genes. In the case of Ford's Yellow Underwings, things are a little more complicated, and very illuminating. The curtisii gene is a 'switch gene' which has what looks like the same effect on both Barra and Orkney, but it achieves it by switching on different suites of genes on the different islands. We notice this only when the two populations are cross-bred. The curtisii switch gene finds itself in a genetic climate which is neither one thing nor the other. It is a mixture of Barra genes and Orkney genes, and the colour pattern that either suite could produce, on its own, breaks down.
What is interesting about this is that either the Barra mixture or the Orkney mixture can put together the colour pattern. There is more than one way of achieving the same result. Both of them involve cooperating suites of genes, but they are two different suites and the members of each suite don't cooperate well with the other. I take this to be a model for what often goes on among working genes within any gene pool. In The Selfish Gene, I used a rowing analogy. A crew of eight oarsmen needs to be well coordinated. Eight men who have trained together can expect to work well together. But if you mix four men from one crew with four from another equally good crew, they don't gel: their rowing fails apart. This is analogous to the mixing of two suites of genes which worked well when each was with its previous companions, but whose coordination breaks down when each is pushed into the foreign genetic climate provided by the other.
Now at this point many biologists get carried away and say that natural selection must work at the level of the whole crew as a unit, the whole suite of genes, or the whole individual organism. They are right that the individual organism is a very important unit in the hierarchy of life. And it really does display unitary qualities. (This is less true of plants than of animals, who tend to have a fixed set of parts, all neatly parcelled inside a skin with a discrete, unitary shape. Individual plants are often harder to delimit as they straggle and vegetatively propagate themselves through meadows and undergrowth. ) But, however unitary and discrete an individual wolf or buffalo, say, may be, the package is temporary and it is unique. Successful buffaloes don't duplicate themselves around the world in the form of multiple copies, they duplicate their genes. The true unit of natural selection has to be a unit of which you can say it has a frequency. It has a frequency which goes up when its type is successful, down when it fails. This is exactly what you can say of genes in gene pools. But you can't say it of individual buffaloes. Successful buffaloes don't become more frequent. Each buffalo is unique. It has a frequency of
one. You can define a buffalo as successful if its genes increase in frequency in future populations.
Field Marshal Montgomery, never the humblest of men, was once heard to remark, 'Now God said (and I agree with Him) . . . ' I feel a bit like that when I read of God's covenant with Abraham. He didn't promise Abraham eternal life as an individual (though Abraham was only 99 at the time, a spring chicken by Genesis standards). But he did promise him something else.
And I will make my covenant between me and thee, and will multiply thee exceedingly . . . and thou shalt be a father of many nations . . . And I will make thee exceeding fruitful, and I will make nations of thee, and kings shall come out of thee. (Genesis 17
Abraham was left in no doubt that the future lay with his seed, not his individuality. God knew his Darwinism.
To resume, the point I am making is that genes, for all that they are the separate units naturally selected in the Darwinian process, are highly cooperative. Selection favours or disfavours single genes for their capacity to survive in their environment, but the most important part of that environment is the genetic climate furnished by other genes. The consequence is that cooperating suites of genes come together in gene pools. Individual bodies are as unitary and coherent as they are, not because natural selection chooses them as units, but because they are built by genes that have been selected to cooperate with other members of the gene pool. They cooperate specifically in the enterprise of building individual bodies. But it is an anarchistic, 'each gene for itself kind of cooperation.
The cooperation, indeed, breaks down whenever the chance arises, as in so-called segregation distorter' genes. There is a gene in mice known as the t gene. In double dose t causes sterility or death, and there must be strong natural selection against it. But in single dose in males, it has a very odd effect. Normally, each copy of a gene should find itself in 50 per cent of the sperms made by a male. I have brown eyes like my mother, but my father has blue, so I know that I carry one copy of the gene for blue eyes and 50 per cent of my sperms carry the blue-eyed gene. In male mice, t doesn't behave in this orderly way. More than 90 per cent of an affected male's sperms contain t. Distorting sperm production is what the t gene does. It is its equivalent of making brown eyes or curly hair. And you can see that, in spite of lethality in double dose, once t arises in a population of mice, it will tend to spread because of its huge success in getting itself into sperms. It has been suggested that outbreaks of t arise in wild populations of mice, spreading like a sort of population cancer
and eventually driving the local population extinct, t is an illustration of what can happen when cooperation among genes breaks down. 'The exception that proves the rule' is often a rather silly expression, but this is a rare occasion when it is appropriate.
To repeat, the main suites of cooperating genes are the whole gene pools of species. Cheetah genes cooperate with cheetah genes but not with camel genes, and vice versa. This is not because cheetah genes, even in the most poetic sense, see any virtue in the preservation of the cheetah species. They are not working to save the cheetah from extinction like some molecular World Wildlife Fund. They are simply surviving in their environment, and their environment largely consists of other genes from the cheetah gene pool. Therefore, abilities to cooperate with other cheetah genes (but not with camel genes or codfish genes) are among the main qualities favoured in the struggle between rival cheetah genes. Just as, in arctic climates, genes for withstanding the cold come to predominate, so, in cheetah gene pools, do genes that are equipped to flourish in the climate of other cheetah genes. As far as each gene is concerned, the other genes in its gene pool are just another aspect of the weather.
The level at which the genes constitute 'weather' for each other is mostly buried in cellular chemistry. Genes code the production of enzymes, protein molecules which work as machine tools churning out one particular component in a chemical production line. There are alternative chemical pathways to the same end, which means alternative production lines. It may not matter greatly which of two production lines is adopted, so long as the cell doesn't attempt both at once. Either of the two production lines might be equally good, but intermediate products yielded by production line A can't be used by production line B, and vice versa. Once again, it is tempting to say that the entire production line is naturally selected, as a unit. This is wrong. What is naturally selected is each individual gene, against the background or climate provided by all the other genes. If the population happens to be dominated by genes for all but one of the steps in production line A, this constitutes a chemical climate in which the gene for the missing A step is favoured. Conversely, a pre-existing climate of B genes favours B genes over A genes. We aren't talking about which is 'better', as though there were some kind of contest between production line A and production line B. What we are saying is that either of the two is fine, but a mixture is unstable. The population has two alternative stable climates of mutually cooperating genes and natural selection will tend to steer the population towards whichever of the two stable states it is already closest to.
But we don't have to talk of biochemistry. We can use the metaphor of genetic climate at the level of organs and behaviour. A cheetah is a
beautifully integrated killing machine, equipped with long, muscled legs and a sinuously sprung backbone for outrunning prey, powerful jaws
and dagger teeth for stabbing them, forward-focused eyes for aiming at them, short guts with appropriate enzymes for digesting them, a brain pre-loaded with carnivorous behaviour software, and collections of other features that make it a typical hunter. On the other side of the arms race, antelopes are equivalently well equipped to eat plants and avoid being caught by predators. Long guts, complicated by blind alleys stuffed with cellulose-digesting bacteria, go with flat grinding teeth, go with brains pre-programmed to alarm and rapid escape, go with exquisitely camouflaged dappling of the pelt. These are two alternative ways of making a living. Neither is obviously better than the other, but either is better than an uneasy compromise: carnivorous guts combined with herbivorous teeth, say, or carnivorous pursuit instincts combined with herbivorous digestive enzymes.
Yet again, it is tempting to speak of the 'whole cheetah' or the 'whole antelope' as being selected 'as a unit'. Tempting, but superficial. Also lazy. It requires some extra thinking work to see what is really going on. Genes that programme the development of carnivorous guts flourish in a
genetic climate that is already dominated by genes programming carnivorous brains. And vice versa. Genes that programme defensive camouflage flourish in a genetic climate that is already dominated by genes programming herbivorous teeth. And vice versa. There are lots and lots of ways of making a living. To mention only a few mammal examples, there is the cheetah way, the impala way, the mole way, the baboon way, the koala way. There is no need to say that one way is better than any other. All of them work. What is bad is to be caught with half your adaptations aimed at one way of life, half aimed at another.
This kind of argument is best expressed at the level of the separate genes. At each genetic locus, the gene most likely to be favoured is the one that is compatible with the genetic climate afforded by the others, the one
that survives in that climate through repeated generations. Since this applies to each one of the genes that constitute the climate - since every gene is potentially part of the climate of every other - the result is that a species gene pool tends to coalesce into a gang of mutually compatible partners. Sorry to go on about this, but some of my respected colleagues refuse to get the point, obstinately insisting that the 'individual' is the 'true' unit of natural selection!
More widely, the environment in which a gene has to survive includes the other species with which it comes into contact. The DNA of any one species doesn't literally come into direct contact with the DNA molecules of its predators, competitors or mutualistic partners. 'Climate' has to be understood less intimately than when the arena of gene cooperation is
the interior of cells, as it is for genes within one species. In the larger arena, it is the consequences of genes in other species - their 'phenotypic effects' - that constitute an important part of the environment in which the natural selection of genes within neighbouring species goes on. A rainforest is a special kind of environment, fashioned and defined by the plants and animals that live in it. Every one of the species in a tropical rainforest consists of a gene pool, isolated from all other gene pools as far as sexual mixing is concerned, but in contact with their bodily effects.
Within each of those separate gene pools, natural selection favours those genes that cooperate within their own gene pool, as we have seen. But it also favours those genes that are good at surviving alongside the consequences of the other gene pools in the rainforest - the trees, vines, monkeys, dung beetles, wood lice and soil bacteria. In the long run this may make the whole forest look like a single harmonious whole, with each unit pulling for the benefit of all, every tree and every soil mite, even every predator and every parasite, playing its part in one big, happy family. Once again, this is a tempting way of looking at it. Once again, it is lazy - bad poetic science. A much truer vision, still poetic science but (it is the purpose of this chapter to persuade you) good poetic science, sees the forest as an anarchistic federation of selfish genes, each selected as being good at surviving within its own gene pool against the background of the environment provided by all the others.
Yes, there is a wishy-washy sense in which organisms in a rainforest perform a valuable service for other species, and even for the maintenance of the whole forest community. Certainly, if you removed all the soil bacteria, the consequences for the trees and ultimately for most of the life of the forest, would be dire. But that is not why the soil bacteria are there. Yes, of course they do break down the dead leaves, dead animals and manure into compost which is useful for the continued prosperity of the whole forest. But they aren't doing it for the sake of making compost. They are using the dead leaves and dead animals as food for themselves, for the good of the genes that programme their compost-making activities. It is an incidental consequence of this self- interested activity that the soil is improved from the point of view of the plants, and therefore the herbivores that eat them, and therefore the carnivores that eat the herbivores. Species in a rainforest community flourish in the presence of the other species in that community because the community is the environment in which their ancestors survived. Maybe there are plants that flourish in the absence of a rich culture of soil bacteria, but those are not the plants we find in a rainforest. We are more likely to find them in a desert.
This is the right way to handle the temptation of 'Gaia': the overrated romantic fancy of the whole world as an organism; of each species doing
its bit for the welfare of the whole; of bacteria, for example, working to improve the gas content of the earth's atmosphere for the good of all life. The most extreme example I know of this kind of bad poetic science comes from a famous and senior 'ecologist' (the quotation marks denote an activist for green politics, rather than a genuine scholar of the academic subject of ecology). It was reported to me by Professor John Maynard Smith, who was attending a conference sponsored by the Open University in Britain. The conversation turned to the mass extinction of the dinosaurs and whether this catastrophe was caused by a cometary collision. The bearded ecologist was in no doubt. 'Of course not,' he said decisively, Gaia would not have permitted it! '
Gaia was the Greek earth goddess whose name has been adopted by James Lovelock, an English atmospheric chemist and inventor, to personify his poetic notion that the whole planet should be regarded as a single living thing. All living creatures are Gaia's body parts and they work together as a well-adjusted thermostat, reacting to perturbations so as to preserve all life. Lovelock is avowedly embarrassed by those, like the ecologist I have just quoted, who take his idea right over the top. Gaia has become a cult, almost a religion, and Lovelock now understandably wants to distance himself from this. But some of his own early suggestions, when you think them through, are only slightly more realistic. He proposed, for instance, that bacteria produce methane gas because of the valuable role it plays in regulating the chemistry of the earth's atmosphere. The problem with this is that individual bacteria are asked to be nicer than natural selection can explain. The bacteria are supposed to produce methane beyond their own needs. They are expected to produce enough methane to benefit the planet in general. It is no good pleading that this is in their own long-term interests because if the planet goes extinct so will they. Natural selection is never aware of the long-term future. It is not aware of anything. Improvements come about not through foresight but by genes coming to outnumber their rivals in gene pools. Unfortunately, genes that make rebel bacteria sit back and enjoy the benefits of their rivals' altruistic production of methane are bound to prosper at the expense of the altruists. So the world will become relatively more full of selfish bacteria. This will continue even if, because of their selfishness, the total number of bacteria (and of everything else) is going down. It will continue even to the point of extinction. How should it not? There is no foresight.
If Lovelock were to retort that the bacteria produce methane as a by- product of something else that they do for their own good, and it is only incidentally useful for the world, I should agree wholeheartedly. But in that case the whole rhetoric of Gaia is superfluous and misleading. You don't need to talk about bacteria working for the good of anything other than their own short-term genetic good. We are left with the conclusion
that individuals work for Gaia only when it suits them to do so - so why bother to bring Gaia into the discussion? We are better off thinking about genes, which are the real, self-replicating units of natural selection, flourishing in an environment which includes the genetic climate furnished by the other genes. I am quite happy to generalize the notion of the genetic climate to include all the genes in the whole world. But that is not Gaia. Gaia falsely focuses attention on planetary life as a single unit. Planetary life is a shifting pattern of genetic weather.
Lovelock's main comrade-in-arms as a champion of Gaia is the American bacteriologist Lynn Margulis. Despite her pugnacious disposition, she places herself firmly on the gentle side of the continuum which I am attacking as bad poetic science. Here she is, writing with her son Dorion Sagan:
Next, the view of evolution as chronic bloody competition among individuals and species, a popular distortion of Darwin's notion of 'survival of the fittest,' dissolves before a new view of continual cooperation, strong interaction, and mutual dependence among life forms. Life did not take over the globe by combat, but by networking. Life forms multiplied and complexified by co-opting others, not just by killing them. Microcosmos: Four Billion Years
of Microbial Evolution (1987)
Margulis and Sagan are in a superficial sense not too far from being right here. But they are misled by bad poetic science into expressing it wrongly. As I emphasized at the beginning of this chapter, the opposition 'combat versus cooperation' is the wrong dichotomy to stress. There is fundamental conflict at the level of the genes. But since the
environments of genes are dominated by each other, cooperation and 'networking' arise automatically as a favoured manifestation of that conflict.
Where Lovelock is a student of the world's atmosphere, Margulis approaches from the other direction, as a specialist in bacteria.
She rightly grants bacteria centre stage among life forms on our planet. At the level of biochemistry, there is a range of fundamental ways of making a living. These are practised by one or another kind of bacteria. One of these basic life recipes has been adopted by eukaryotes (that's everybody except bacteria), and we get it from bacteria. Margulis has successfully argued over many years that most of our biochemistry is carried out for us by what were once free bacteria now living within our cells. Here's another quotation from the same book by Margulis and Sagan.
Bacteria, by contrast, exhibit a far wider range of metabolic variations than eukaryotes. They indulge in bizarre fermentations, produce
methane gas, 'eat' nitrogen gas right out of the air, derive energy from globules of sulfur, precipitate iron and manganese while breathing, combust hydrogen using oxygen to make water grow in boiling water and in salt brine, store energy by use of the purple pigment rhodopsin, and so forth . . . We, however, use just one of their many metabolic designs for energy production, namely that of aerobic respiration, the specialty of mitochondria.
Aerobic respiration, an elaborate set of biochemical cycles and chains whereby energy trapped from the sun is released from organic molecules, goes on in the mitochondria, the minute organelles that swarm inside our cells. Margulis has convinced the scientific world, rightly I think, that mitochondria are descended from bacteria. The ancestors of mitochondria, when they lived on their own, evolved the biochemical tricks that we call aerobic respiration. We eucaryotes now benefit from this advanced chemical wizardry because our cells contain the descendants of the bacteria that discovered them. On this view there is an unbroken line of descent from modern mitochondria back to ancestral bacteria living free in the sea. When I say 'line of descent', I literally mean that a free-living bacterial cell divided into two, and at least one of those divided into two, and at least one of those divided into two and so on until we reach every one of your mitochondria, continuing to divide in your cells.
Margulis believes that mitochondria were originally parasites (or predators - the distinction is not important at this level) which attacked the larger bacteria that were destined to provide the shell of the eucaryotic cell. There are still some bacterial parasites that do a similar trick, burrowing through the prey's cell wall, then, when safely inside, sealing up the wall and eating the cell from within. The mitochondrial ancestors, according to the theory, evolved from parasites that kill to less virulent parasites that keep their host alive to exploit it longer. Later still, the host cells began to benefit from the metabolic activities of the proto- mitochondria. The relationship shifted from predatory or parasitic (good for one side, bad for the other) to mutualistic (good for both). As the mutualism deepened, each came to depend more thoroughly on the other, and each came to lose those bits of itself whose purpose was best served by the other.
In a Darwinian world, such dedicatedly intimate cooperation evolves only when the DNA of the parasite passes 'longitudinally' down host generations in the same vehicles as the DNA of the host. To this day, our mitochondria still have their own DNA, which is only distantly related to our 'own' DNA and more closely related to that of certain bacteria. But it passes down human generations in human eggs. Parasites whose DNA passes longitudinally like this (that is, from host parent to host child)
become less virulent and more cooperative, because anything that is
good for the host DNA's survival automatically tends to be good for their own DNA's survival. Parasites whose DNA passes 'horizontally' (from a host to some other host which is not specifically its own child), for example rabies or flu viruses, may become even more virulent. If DNA is to be transmitted horizontally, the death of the host may be no bad thing. An extreme might be a parasite that feeds inside an individual host, turning its flesh into spores until it finally bursts, scattering the parasite DNA to the winds where it is blown far and wide to find new hosts.
Mitochondria are extreme longitudinal specialists. So intimate with the host cells did they become that it is hard for us to recognize that they were ever apart. My Oxford colleague Sir David Smith has found a neat simile:
In the cell habitat, an invading organism can progressively lose pieces of itself, slowly blending into the general background, its former existence betrayed only by some relic. Indeed, one is reminded of Alice in Wonderland's encounter with the Cheshire Cat. As she watched it, "it vanished quite slowly, beginning with the tail, and ending with the grin, which remained some time after the rest of it had gone". There are a number of objects in a cell like the grin of the Cheshire Cat. For those who try to trace their origin, the grin is challenging and truly enigmatic. The Cell as a Habitat (1979)
I don't find any strong distinction between the relationship of mitochondrial DNA to host DNA and that between one gene and another within the normal orthodox gene pool of a species 'own' genes. I have argued that all our 'own' genes should be seen as mutually parasitic upon each other.
The other grinning relic which is now pretty uncontroversial is the chloroplast. Chloroplasts are small bodies in plant cells that do the business of photosynthesis - storing solar energy by using it to synthesize organic molecules. These organic molecules can then be broken down later and the energy released in a controlled way when required. Chloroplasts are responsible for the green colour of plants. It is now widely agreed that they are descended from photosynthetic bacteria, cousins of the 'blue-green' bacteria that still float free today and are responsible for 'blooms' in polluted water. The process of photosynthesis is the same in these bacteria and in (the chloroplasts of) eucaryotes. Chloroplasts, according to Margulis, were captured in a different way from mitochondria. Where the mitochondrial ancestors aggressively invaded larger hosts, the ancestors of chloroplasts were prey, originally engulfed for food, only later evolving a mutualistic rapport with their
captors, doubtless again because their DNA became transmitted longitudinally down host generations.
More controversially, Margulis believes that yet another kind of bacteria, the spirally moving spirochaetes, invaded the early eucaryotic cell and contributed such moving structures as cilia, flagella and the 'spindles' which drag the chromosomes apart in cell division. Cilia and flagella (singular, cilium and flagellum) are just different-sized versions of each other, and Margulis prefers to call both 'undulipodia'. She reserves the name flagellum for the superficially similar but actually very different whip-like structure that some bacteria use to paddle ('screw' is a more appropriate verb) themselves along. The bacterial flagellum, incidentally, is remarkable in having the only true rotating bearing in the living kingdoms. It is nature's only important example of 'the wheel', or at least the axle, before humans reinvented it. Cilia and other eucaryotic undulipodia are more complicated. Margulis identifies each individual undulipodium with an entire spirochaete bacterium, in the same way as she identifies each mitochondrion and each chloroplast with an entire bacterium.
The idea of co-opting bacteria to perform some difficult biochemical trick has frequently resurfaced in more recent evolution. Deep sea fish have luminous organs to signal to each other and even to find their way about. Rather than undertake the difficult chemical task of making light, they have co-opted bacteria that specialize in the skill. The luminous organ of a fish is a bag of carefully cultured bacteria, which give off light as a spin off from their own biochemical purposes.
So we have a whole new way of looking at an individual organism. Not only do animals and plants participate in complicated webs of interaction with each other, and with individuals of other species, in populations and communities like a tropical rainforest or a coral reef. Each individual animal or plant is a community. It is a community of billions of cells, and each one of those billions of cells is a community of thousands of bacteria. I'd go further and say that even a species' 'own' genes are a community of selfish co-operators. Now we are tempted by yet another piece of poetic science, the poetry of hierarchy. There are units within larger units, not just up to the level of the individual organism but even higher, for organisms live in communities. Is there not, at every level in the hierarchy, symbiotic cooperation between units at the next level down, units that used to be independent?
Perhaps there is some mileage in this. Termites make a very successful living out of eating wood and wood products such as books. But, yet again, the necessary chemical tricks do not come naturally to the termite's own cells. Just as the unaided eucaryote cell has to borrow the
biochemical talents of mitochondria, so termite guts, on their own, cannot digest wood. They rely on symbiotic micro-organisms to do the business of digesting the wood. The termite itself subsists on the micro- organisms and their excreta. These micro organisms are strange and specialized creatures, mostly' found nowhere else in the world but in the guts of their own species of termite. They depend on the termites (to find the wood and chew it physically into small pieces), just as the termites depend on them (to break it down to even smaller molecular pieces, using enzymes which the termites themselves can't make). Some of the micro-organisms are bacteria, some of them are protozoa (single-celled eucaryotes), and some of them are a fascinating mixture of the two. Fascinating because of a kind of evolutionary deja vu which powerfully adds plausibility to Margulis's speculation.
Mixotricha paradoxa is a flagellate protozoan which lives in the guts of the Australian termite Mastotermes darwiniensis. It has four large cilia at the front end. Margulis, of courses believes that these are themselves originally derived from symbiotic spirochaetes. But, though that may be controversial, there is a second kind of small, waving, hair-like projection about which there is no doubt. Covering the rest of the body, these look like cilia: cilia such as those that beat rhythmically to waft our eggs down our oviducts. But they are not cilia. Every one of them - and there are about half a million - is a tiny spirochaete bacterium. Indeed, there are two quite different kinds of spirochaete involved. It is these waving bacteria that propel Mixotricha around in the termite gut, and it is reported that they wave in unison. This seems hard to believe until you realize that each one could simply be provoked by its immediate neighbours.
The four large cilia at the front seem to serve only as rudders. These might be described as Mixotricha's 'own', to distinguish them from the spirochaetes that carpet the rest of the body. But of course, if Margulis is right, they are really no more Mixotricha's own than the spirochaetes: they just represent an earlier invasion. The deja vu lies in the re- enactment by new spirochaetes, of a drama that was first staged a billion years ago. As it happens, Mixotricha cannot use oxygen because there isn't enough of it in the termite gut. Otherwise, we may be sure, they'd have mitochondria inside them - relic of yet another ancient wave of bacterial invasion. But in any case they do have other symbiotic bacteria inside them which are probably performing a biochemical role rather like mitochondria, maybe assisting with the difficult task of digesting wood.
A single Mixotricha individual, therefore, is a colony containing at least half a million symbiotic bacteria of various kinds. From a functional point of view as a wood-digesting machine, a single termite is a colony of perhaps as many symbiotic micro-organisms in its gut. Don't forget that,
quite apart from the 'recent' invaders of its gut flora, a termite's 'own' cells, like the cells of any other eucaryote, are themselves colonies of much older bacteria. Finally, termites are rather special in that they themselves live in massive colonies of mostly sterile worker insects which plunder the country side more effectively than almost any other kind of animal except ants - and they are successful for the same kind of reason. Mastotermes colonies can contain up to a million individual worker termites. The species is a voracious pest in Australia, devouring telegraph poles, the plastic lining of electric cables, wooden buildings and bridges, even billiard balls. Being a colony of colonies of colonies seems to be a successful recipe for life.
I want to return to the genes' eye view and push the idea of universal symbiosis - 'living together' - to its ultimate conclusion. Margulis is rightly seen as a high priestess of symbiosis. As I said earlier, I would go even further, and regard all 'normal' nuclear genes as symbiotic in the same kind of way as mitochondrial genes. But where Margulis and Lovelock invoke the poetry of cooperation and amity as primary in the union, I want to do the opposite and regard it as a secondary consequence. At the genetic level all is selfish, but the selfish ends of genes are served by cooperation at many levels. As far as the genes themselves are concerned, the relationships among our 'own' genes are not, in principle, different from the relationship between our genes and mitochondrial genes, or our genes and those of other species. All genes are being selected for their capacity to flourish in the presence of the other genes - of whatever species - whose consequences surround them.
Collaboration within gene pools to make complex bodies is often called co-adaptation, as distinct from co-evolution. Co-adaptation usually refers to the mutual tailoring of different bits of the same kind of organism to other bits. For example, many flowers have both a bright colour to attract insects and dark lines that act as runway guides to lead insects towards the nectar. Colour, lines and nectaries assist each other. They are co- adapted to each other, the genes that make them being selected in each others presence. Co-evolution is normally used to mean mutual evolution in different species. The flowers and the insects that pollinate them evolve together - co-evolve. In this case the co-evolutionary relationship is mutually beneficial. The word co-evolution is also used for the ' hostile kind of evolving together - co-evolutionary 'arms races'.
High-speed running in predators co-evolves with high-speed running in their prey. Thick armour co-evolves with weapons and techniques for penetrating it.
Although I have just made a clear distinction between 'within species' co- adaptation and 'between species' co-evolution, we can now see that a certain amount of confusion is pardonable. If we take the view, as I have
in this chapter, that gene interactions are just gene interactions, at any level, co-adaptation turns out to be just a special case of co-evolution. As far as the genes themselves are concerned, 'within species' is not fundamentally different from 'between species'. The differences are practical. Within a species, genes meet their companions inside cells. Between species, their consequences in the outside world may meet the consequences of the other genes, out in the external world. Intermediate cases, such as intimate parasites and mitochondria, are revealing because they blur the distinction.
Sceptics of natural selection often worry along the following lines. Natural selection, they say, is a purely negative process. It weeds out the unfit. How can such a negative weeding-out play the positive role of building up complex adaptation? A large part of the answer lies in a combination of co-evolution and co-adaptation, two processes which, as we have just seen, are not so very far apart.
Co-evolution, like a human arms race, is a recipe for progressive build- up of improvements (I mean improvements in efficiency at what they do, of course; obviously, from a humane point of view, 'improvements' in armaments are just the reverse). If predators get better at their job, prey have to follow suit just to stay in the same place. And vice versa. The same goes for parasites and hosts. Escalation begets further escalation. This leads to real progressive improvement in equipment for survival, even if it does not lead to improvement in survival itself (because, after all, the other side in the arms race is improving too). So, co-evolution - arms races, the mutual evolution of genes in different gene pools - is one answer to the sceptic who thinks natural selection is a purely negative process.
The other answer is co-adaptation, the mutual evolution of genes in the same gene pool. In the cheetah gene pool, carnivorous teeth work best with carnivorous guts and carnivorous habits. Herbivorous teeth, guts and habits form an alternative complex in an antelope gene pool. At the gene level, as we have seen, selection puts together harmonious complexes, not by choosing whole complexes but by favouring each part of the complex within gene pools that are dominated by the other parts of the complex. In the shifting balance of gene pools, more than one stable solution to the same problem may exist. Once a gene pool starts to become dominated by one stable solution, further selection of selfish genes favours the ingredients of the same solution. The other solution could equally well have been favoured if the starting conditions had been different. In any case, the sceptic's worry about whether natural selection is purely a negative, subtracting process is disarmed. Natural selection is positive and constructive. It is no more negative than a sculptor subtracting marble from a block. It carves out of gene pools complexes of
mutually interacting, co-adapted genes: fundamentally selfish but pragmatically cooperating. The unit that the Darwinian sculptor carves is the gene pool of a species.
I have devoted space in the last couple of chapters to warning of bad poetry in science. But the balance of my book is the opposite. Science is poetic, ought to be poetic, has much to learn from poets and should press good poetic imagery and metaphor into its inspirational service. 'The selfish gene' is a metaphoric image, potentially a good one but capable of sadly misleading if the metaphor of personification is improperly grasped. If interpreted aright it may lead us into paths of deep understanding and fertile research. This chapter has used the metaphor of the personified gene to explain a sense in which 'selfish' genes are also 'cooperative'.
The key image to be floated in the next chapter is that of a species' genes as a detailed description of the collection of environments in which its ancestors lived - a genetic book of the dead.
10
THE GENETIC BOOK OF THE DEAD
Remember the wisdom out of the old days . . .
W. B. YEATS, The Wind Among the Reeds (1899)
The first essay I can remember writing at school was 'The Diary of a Penny'. You had to imagine yourself a coin and tell your story, of how you sat in a bank for a while until you were given out to a customer, how it felt to jangle around in his pocket with the other coins, how you were handed over to buy something, then how you were passed out as change to another customer and then . . . well, you probably wrote a similar essay yourself. It is helpful to think the same way for a gene travelling not from pocket to pocket but from body to body down the generations. And the first point that the analogy of the coin makes is that of course the personification of the gene is not to be taken literally, any more than we seven-year-olds really thought our coins could talk. Personification is sometimes a useful device, and for critics to accuse us of taking it literally is almost as stupid as taking it literally in the first place. Physicists are not literally charmed by their particles, and the critic who would so accuse them is a tiresome pedant.
The 'minting' event for a gene is the mutation that brought it into existence by altering a previous gene. Only one of many copies of the gene in the population is changed (by one mutation event, but an
identical mutation may change another copy of the gene in the gene pool at another time). The others continue to make copies of the original gene, which may now be said to be in competition with the mutant form. Making copies is of course what genes, unlike coins, are supremely good at, and our diary of a gene has to include the experiences not of the particular atoms that go to make up the DNA, but the DNA's experiences in the form of multiple copies in successive generations. As the last chapter showed, much of a gene's 'experience' of past generations consists of rubbing up against other genes of the species, and this is why they cooperate so amicably in the collective enterprise of building bodies.
Now let's ask the question whether all the genes of a species have the same ancestral 'experiences'. Mostly they do. Most buffalo genes can look back to a long line of buffalo bodies which enjoyed, or suffered, common buffalo experiences. The bodies in which these genes survived included male and female buffaloes, large and small, and so on. But there are subsets of genes with different experiences, for example the genes that determine sex. In mammals, Y chromosomes are found only in males and do not exchange genes with other chromosomes. So a gene sitting on a Y chromosome has had a limited experience of buffalo bodies: male ones only. Its experiences are largely typical of buffalo genes in general, but not entirely so. Unlike most buffalo genes it doesn't know what it is like to sit in a female buffalo. A gene that has always been on a Y chromosome since the origin of the mammals during the age of dinosaurs will have experienced male bodies of many different species, but never a female body of any kind. The case of X chromosomes is more complicated to work out. Male mammals have one X chromosome (inherited from the mother, plus one Y chromosome inherited from the father), while females have two X chromosomes (one from each parent). So each X chromosome gene has experienced both female and male bodies, but two-thirds of its experience has been in female bodies. In birds the situation is reversed. The female bird has uneven sex chromosomes (which we may as well call X and Y by analogy with mammals, although the official bird terminology is different), the male two of the same (XX). The genes on the other chromosomes have all had an equal experience of male and female bodies, but their experiences may be unequal in other respects. A gene will have spent more than its fair share of time in ancestral bodies that possess whatever qualities the gene encodes - long legs, thick horns, or whatever it may be, especially if it is a dominant gene. Almost as obviously, all genes are likely to have spent more of their ancestral time in successful than in unsuccessful bodies. There are plenty of unsuccessful bodies about and they contain their full complement of genes. But they tend not to have descendants (that is what being unsuccessful means) so, as a gene looks back through its biography of past bodies, it will observe that all of them were as a matter of fact successful (by definition), and perhaps most (but not
all) of them were equipped with what it normally takes to be successful. The difference here is that individuals who are not equipped to be successful sometimes reproduce despite their lack. And individuals who are superbly equipped to survive and reproduce under average conditions are sometimes struck by lightning.
If, like some deer, seals and monkeys, the species is one in which the males form dominance hierarchies and dominant males do most of the reproducing, it will follow that the genes of the species will have more experience of dominant male bodies than of subordinate ones. (Note that we are no longer using dominant in its technical genetic sense, whose opposite is recessive, but in its ordinary language sense, where the opposite is subordinate. ) In every- generation, most of the males are subordinate, but their genes still look back on a strong line of dominant male ancestors. In every generation, the majority are fathered by a dominant minority from the previous generation.
I first understood this while listening to lectures by the late E. B. Ford, legendary Oxford aesthete and eccentric founder of the now neglected school of Ecological Geneticists. Most of Ford's research was on wild populations of butterflies and moths. Among these was the Lesser Yellow Underwing moth, Triphaena comes. This moth is normally yellowish brown, but there is a variant called curtisii which is blackish. Curtisii is not found in England at all; however, in Scotland and the Isles curtisii coexists with the normal comes. The curtisii dark colour pattern is nearly completely dominant to the normal comes pattern. 'Dominant to' is a technical term, which is why I can't just say 'dominates'. It means that hybrids between the two look like curtisii even though they bear the genes of both. Ford caught specimens from Barra in the Outer Hebrides, west of Scotland, and from one of the Orkney islands, north of Scotland, as well as from the Scottish mainland itself. Each of the two island forms looks exactly like its opposite number at the other island site, and the dark curtisii gene is dominant on the two islands, as well as on the mainland. Other evidence shows that the curtisii gene is the very same gene in all localities. In view of this you'd expect that, when you cross- bred specimens from different islands, the normal dominance pattern would hold up. But it doesn't, and this is the point of the story. Ford caught individuals from Barra and mated them with individuals from Orkney. And the dominance of curtisii completely disappeared. A full range of intermediates turned up in the hybrid families, just as if there was no dominance. What seems to be going on is this. The curtisii gene does not, in itself, encode the formula for the coloured pigment by which we distinguish the moths, nor is dominance ever a property of a gene on its own. Instead, like any other gene, the curtisii gene should be thought
of as having its effects only in the context of a suite of other genes, some of which it 'switches on'. This suite of other genes is part of what I mean by 'genetic background' or 'genetic climate'. In theory, any gene could therefore exert radically different effects on different islands, in the presence of different suites of other genes. In the case of Ford's Yellow Underwings, things are a little more complicated, and very illuminating. The curtisii gene is a 'switch gene' which has what looks like the same effect on both Barra and Orkney, but it achieves it by switching on different suites of genes on the different islands. We notice this only when the two populations are cross-bred. The curtisii switch gene finds itself in a genetic climate which is neither one thing nor the other. It is a mixture of Barra genes and Orkney genes, and the colour pattern that either suite could produce, on its own, breaks down.
What is interesting about this is that either the Barra mixture or the Orkney mixture can put together the colour pattern. There is more than one way of achieving the same result. Both of them involve cooperating suites of genes, but they are two different suites and the members of each suite don't cooperate well with the other. I take this to be a model for what often goes on among working genes within any gene pool. In The Selfish Gene, I used a rowing analogy. A crew of eight oarsmen needs to be well coordinated. Eight men who have trained together can expect to work well together. But if you mix four men from one crew with four from another equally good crew, they don't gel: their rowing fails apart. This is analogous to the mixing of two suites of genes which worked well when each was with its previous companions, but whose coordination breaks down when each is pushed into the foreign genetic climate provided by the other.
Now at this point many biologists get carried away and say that natural selection must work at the level of the whole crew as a unit, the whole suite of genes, or the whole individual organism. They are right that the individual organism is a very important unit in the hierarchy of life. And it really does display unitary qualities. (This is less true of plants than of animals, who tend to have a fixed set of parts, all neatly parcelled inside a skin with a discrete, unitary shape. Individual plants are often harder to delimit as they straggle and vegetatively propagate themselves through meadows and undergrowth. ) But, however unitary and discrete an individual wolf or buffalo, say, may be, the package is temporary and it is unique. Successful buffaloes don't duplicate themselves around the world in the form of multiple copies, they duplicate their genes. The true unit of natural selection has to be a unit of which you can say it has a frequency. It has a frequency which goes up when its type is successful, down when it fails. This is exactly what you can say of genes in gene pools. But you can't say it of individual buffaloes. Successful buffaloes don't become more frequent. Each buffalo is unique. It has a frequency of
one. You can define a buffalo as successful if its genes increase in frequency in future populations.
Field Marshal Montgomery, never the humblest of men, was once heard to remark, 'Now God said (and I agree with Him) . . . ' I feel a bit like that when I read of God's covenant with Abraham. He didn't promise Abraham eternal life as an individual (though Abraham was only 99 at the time, a spring chicken by Genesis standards). But he did promise him something else.
And I will make my covenant between me and thee, and will multiply thee exceedingly . . . and thou shalt be a father of many nations . . . And I will make thee exceeding fruitful, and I will make nations of thee, and kings shall come out of thee. (Genesis 17
Abraham was left in no doubt that the future lay with his seed, not his individuality. God knew his Darwinism.
To resume, the point I am making is that genes, for all that they are the separate units naturally selected in the Darwinian process, are highly cooperative. Selection favours or disfavours single genes for their capacity to survive in their environment, but the most important part of that environment is the genetic climate furnished by other genes. The consequence is that cooperating suites of genes come together in gene pools. Individual bodies are as unitary and coherent as they are, not because natural selection chooses them as units, but because they are built by genes that have been selected to cooperate with other members of the gene pool. They cooperate specifically in the enterprise of building individual bodies. But it is an anarchistic, 'each gene for itself kind of cooperation.
The cooperation, indeed, breaks down whenever the chance arises, as in so-called segregation distorter' genes. There is a gene in mice known as the t gene. In double dose t causes sterility or death, and there must be strong natural selection against it. But in single dose in males, it has a very odd effect. Normally, each copy of a gene should find itself in 50 per cent of the sperms made by a male. I have brown eyes like my mother, but my father has blue, so I know that I carry one copy of the gene for blue eyes and 50 per cent of my sperms carry the blue-eyed gene. In male mice, t doesn't behave in this orderly way. More than 90 per cent of an affected male's sperms contain t. Distorting sperm production is what the t gene does. It is its equivalent of making brown eyes or curly hair. And you can see that, in spite of lethality in double dose, once t arises in a population of mice, it will tend to spread because of its huge success in getting itself into sperms. It has been suggested that outbreaks of t arise in wild populations of mice, spreading like a sort of population cancer
and eventually driving the local population extinct, t is an illustration of what can happen when cooperation among genes breaks down. 'The exception that proves the rule' is often a rather silly expression, but this is a rare occasion when it is appropriate.
To repeat, the main suites of cooperating genes are the whole gene pools of species. Cheetah genes cooperate with cheetah genes but not with camel genes, and vice versa. This is not because cheetah genes, even in the most poetic sense, see any virtue in the preservation of the cheetah species. They are not working to save the cheetah from extinction like some molecular World Wildlife Fund. They are simply surviving in their environment, and their environment largely consists of other genes from the cheetah gene pool. Therefore, abilities to cooperate with other cheetah genes (but not with camel genes or codfish genes) are among the main qualities favoured in the struggle between rival cheetah genes. Just as, in arctic climates, genes for withstanding the cold come to predominate, so, in cheetah gene pools, do genes that are equipped to flourish in the climate of other cheetah genes. As far as each gene is concerned, the other genes in its gene pool are just another aspect of the weather.
The level at which the genes constitute 'weather' for each other is mostly buried in cellular chemistry. Genes code the production of enzymes, protein molecules which work as machine tools churning out one particular component in a chemical production line. There are alternative chemical pathways to the same end, which means alternative production lines. It may not matter greatly which of two production lines is adopted, so long as the cell doesn't attempt both at once. Either of the two production lines might be equally good, but intermediate products yielded by production line A can't be used by production line B, and vice versa. Once again, it is tempting to say that the entire production line is naturally selected, as a unit. This is wrong. What is naturally selected is each individual gene, against the background or climate provided by all the other genes. If the population happens to be dominated by genes for all but one of the steps in production line A, this constitutes a chemical climate in which the gene for the missing A step is favoured. Conversely, a pre-existing climate of B genes favours B genes over A genes. We aren't talking about which is 'better', as though there were some kind of contest between production line A and production line B. What we are saying is that either of the two is fine, but a mixture is unstable. The population has two alternative stable climates of mutually cooperating genes and natural selection will tend to steer the population towards whichever of the two stable states it is already closest to.
But we don't have to talk of biochemistry. We can use the metaphor of genetic climate at the level of organs and behaviour. A cheetah is a
beautifully integrated killing machine, equipped with long, muscled legs and a sinuously sprung backbone for outrunning prey, powerful jaws
and dagger teeth for stabbing them, forward-focused eyes for aiming at them, short guts with appropriate enzymes for digesting them, a brain pre-loaded with carnivorous behaviour software, and collections of other features that make it a typical hunter. On the other side of the arms race, antelopes are equivalently well equipped to eat plants and avoid being caught by predators. Long guts, complicated by blind alleys stuffed with cellulose-digesting bacteria, go with flat grinding teeth, go with brains pre-programmed to alarm and rapid escape, go with exquisitely camouflaged dappling of the pelt. These are two alternative ways of making a living. Neither is obviously better than the other, but either is better than an uneasy compromise: carnivorous guts combined with herbivorous teeth, say, or carnivorous pursuit instincts combined with herbivorous digestive enzymes.
Yet again, it is tempting to speak of the 'whole cheetah' or the 'whole antelope' as being selected 'as a unit'. Tempting, but superficial. Also lazy. It requires some extra thinking work to see what is really going on. Genes that programme the development of carnivorous guts flourish in a
genetic climate that is already dominated by genes programming carnivorous brains. And vice versa. Genes that programme defensive camouflage flourish in a genetic climate that is already dominated by genes programming herbivorous teeth. And vice versa. There are lots and lots of ways of making a living. To mention only a few mammal examples, there is the cheetah way, the impala way, the mole way, the baboon way, the koala way. There is no need to say that one way is better than any other. All of them work. What is bad is to be caught with half your adaptations aimed at one way of life, half aimed at another.
This kind of argument is best expressed at the level of the separate genes. At each genetic locus, the gene most likely to be favoured is the one that is compatible with the genetic climate afforded by the others, the one
that survives in that climate through repeated generations. Since this applies to each one of the genes that constitute the climate - since every gene is potentially part of the climate of every other - the result is that a species gene pool tends to coalesce into a gang of mutually compatible partners. Sorry to go on about this, but some of my respected colleagues refuse to get the point, obstinately insisting that the 'individual' is the 'true' unit of natural selection!
More widely, the environment in which a gene has to survive includes the other species with which it comes into contact. The DNA of any one species doesn't literally come into direct contact with the DNA molecules of its predators, competitors or mutualistic partners. 'Climate' has to be understood less intimately than when the arena of gene cooperation is
the interior of cells, as it is for genes within one species. In the larger arena, it is the consequences of genes in other species - their 'phenotypic effects' - that constitute an important part of the environment in which the natural selection of genes within neighbouring species goes on. A rainforest is a special kind of environment, fashioned and defined by the plants and animals that live in it. Every one of the species in a tropical rainforest consists of a gene pool, isolated from all other gene pools as far as sexual mixing is concerned, but in contact with their bodily effects.
Within each of those separate gene pools, natural selection favours those genes that cooperate within their own gene pool, as we have seen. But it also favours those genes that are good at surviving alongside the consequences of the other gene pools in the rainforest - the trees, vines, monkeys, dung beetles, wood lice and soil bacteria. In the long run this may make the whole forest look like a single harmonious whole, with each unit pulling for the benefit of all, every tree and every soil mite, even every predator and every parasite, playing its part in one big, happy family. Once again, this is a tempting way of looking at it. Once again, it is lazy - bad poetic science. A much truer vision, still poetic science but (it is the purpose of this chapter to persuade you) good poetic science, sees the forest as an anarchistic federation of selfish genes, each selected as being good at surviving within its own gene pool against the background of the environment provided by all the others.
Yes, there is a wishy-washy sense in which organisms in a rainforest perform a valuable service for other species, and even for the maintenance of the whole forest community. Certainly, if you removed all the soil bacteria, the consequences for the trees and ultimately for most of the life of the forest, would be dire. But that is not why the soil bacteria are there. Yes, of course they do break down the dead leaves, dead animals and manure into compost which is useful for the continued prosperity of the whole forest. But they aren't doing it for the sake of making compost. They are using the dead leaves and dead animals as food for themselves, for the good of the genes that programme their compost-making activities. It is an incidental consequence of this self- interested activity that the soil is improved from the point of view of the plants, and therefore the herbivores that eat them, and therefore the carnivores that eat the herbivores. Species in a rainforest community flourish in the presence of the other species in that community because the community is the environment in which their ancestors survived. Maybe there are plants that flourish in the absence of a rich culture of soil bacteria, but those are not the plants we find in a rainforest. We are more likely to find them in a desert.
This is the right way to handle the temptation of 'Gaia': the overrated romantic fancy of the whole world as an organism; of each species doing
its bit for the welfare of the whole; of bacteria, for example, working to improve the gas content of the earth's atmosphere for the good of all life. The most extreme example I know of this kind of bad poetic science comes from a famous and senior 'ecologist' (the quotation marks denote an activist for green politics, rather than a genuine scholar of the academic subject of ecology). It was reported to me by Professor John Maynard Smith, who was attending a conference sponsored by the Open University in Britain. The conversation turned to the mass extinction of the dinosaurs and whether this catastrophe was caused by a cometary collision. The bearded ecologist was in no doubt. 'Of course not,' he said decisively, Gaia would not have permitted it! '
Gaia was the Greek earth goddess whose name has been adopted by James Lovelock, an English atmospheric chemist and inventor, to personify his poetic notion that the whole planet should be regarded as a single living thing. All living creatures are Gaia's body parts and they work together as a well-adjusted thermostat, reacting to perturbations so as to preserve all life. Lovelock is avowedly embarrassed by those, like the ecologist I have just quoted, who take his idea right over the top. Gaia has become a cult, almost a religion, and Lovelock now understandably wants to distance himself from this. But some of his own early suggestions, when you think them through, are only slightly more realistic. He proposed, for instance, that bacteria produce methane gas because of the valuable role it plays in regulating the chemistry of the earth's atmosphere. The problem with this is that individual bacteria are asked to be nicer than natural selection can explain. The bacteria are supposed to produce methane beyond their own needs. They are expected to produce enough methane to benefit the planet in general. It is no good pleading that this is in their own long-term interests because if the planet goes extinct so will they. Natural selection is never aware of the long-term future. It is not aware of anything. Improvements come about not through foresight but by genes coming to outnumber their rivals in gene pools. Unfortunately, genes that make rebel bacteria sit back and enjoy the benefits of their rivals' altruistic production of methane are bound to prosper at the expense of the altruists. So the world will become relatively more full of selfish bacteria. This will continue even if, because of their selfishness, the total number of bacteria (and of everything else) is going down. It will continue even to the point of extinction. How should it not? There is no foresight.
If Lovelock were to retort that the bacteria produce methane as a by- product of something else that they do for their own good, and it is only incidentally useful for the world, I should agree wholeheartedly. But in that case the whole rhetoric of Gaia is superfluous and misleading. You don't need to talk about bacteria working for the good of anything other than their own short-term genetic good. We are left with the conclusion
that individuals work for Gaia only when it suits them to do so - so why bother to bring Gaia into the discussion? We are better off thinking about genes, which are the real, self-replicating units of natural selection, flourishing in an environment which includes the genetic climate furnished by the other genes. I am quite happy to generalize the notion of the genetic climate to include all the genes in the whole world. But that is not Gaia. Gaia falsely focuses attention on planetary life as a single unit. Planetary life is a shifting pattern of genetic weather.
Lovelock's main comrade-in-arms as a champion of Gaia is the American bacteriologist Lynn Margulis. Despite her pugnacious disposition, she places herself firmly on the gentle side of the continuum which I am attacking as bad poetic science. Here she is, writing with her son Dorion Sagan:
Next, the view of evolution as chronic bloody competition among individuals and species, a popular distortion of Darwin's notion of 'survival of the fittest,' dissolves before a new view of continual cooperation, strong interaction, and mutual dependence among life forms. Life did not take over the globe by combat, but by networking. Life forms multiplied and complexified by co-opting others, not just by killing them. Microcosmos: Four Billion Years
of Microbial Evolution (1987)
Margulis and Sagan are in a superficial sense not too far from being right here. But they are misled by bad poetic science into expressing it wrongly. As I emphasized at the beginning of this chapter, the opposition 'combat versus cooperation' is the wrong dichotomy to stress. There is fundamental conflict at the level of the genes. But since the
environments of genes are dominated by each other, cooperation and 'networking' arise automatically as a favoured manifestation of that conflict.
Where Lovelock is a student of the world's atmosphere, Margulis approaches from the other direction, as a specialist in bacteria.
She rightly grants bacteria centre stage among life forms on our planet. At the level of biochemistry, there is a range of fundamental ways of making a living. These are practised by one or another kind of bacteria. One of these basic life recipes has been adopted by eukaryotes (that's everybody except bacteria), and we get it from bacteria. Margulis has successfully argued over many years that most of our biochemistry is carried out for us by what were once free bacteria now living within our cells. Here's another quotation from the same book by Margulis and Sagan.
Bacteria, by contrast, exhibit a far wider range of metabolic variations than eukaryotes. They indulge in bizarre fermentations, produce
methane gas, 'eat' nitrogen gas right out of the air, derive energy from globules of sulfur, precipitate iron and manganese while breathing, combust hydrogen using oxygen to make water grow in boiling water and in salt brine, store energy by use of the purple pigment rhodopsin, and so forth . . . We, however, use just one of their many metabolic designs for energy production, namely that of aerobic respiration, the specialty of mitochondria.
Aerobic respiration, an elaborate set of biochemical cycles and chains whereby energy trapped from the sun is released from organic molecules, goes on in the mitochondria, the minute organelles that swarm inside our cells. Margulis has convinced the scientific world, rightly I think, that mitochondria are descended from bacteria. The ancestors of mitochondria, when they lived on their own, evolved the biochemical tricks that we call aerobic respiration. We eucaryotes now benefit from this advanced chemical wizardry because our cells contain the descendants of the bacteria that discovered them. On this view there is an unbroken line of descent from modern mitochondria back to ancestral bacteria living free in the sea. When I say 'line of descent', I literally mean that a free-living bacterial cell divided into two, and at least one of those divided into two, and at least one of those divided into two and so on until we reach every one of your mitochondria, continuing to divide in your cells.
Margulis believes that mitochondria were originally parasites (or predators - the distinction is not important at this level) which attacked the larger bacteria that were destined to provide the shell of the eucaryotic cell. There are still some bacterial parasites that do a similar trick, burrowing through the prey's cell wall, then, when safely inside, sealing up the wall and eating the cell from within. The mitochondrial ancestors, according to the theory, evolved from parasites that kill to less virulent parasites that keep their host alive to exploit it longer. Later still, the host cells began to benefit from the metabolic activities of the proto- mitochondria. The relationship shifted from predatory or parasitic (good for one side, bad for the other) to mutualistic (good for both). As the mutualism deepened, each came to depend more thoroughly on the other, and each came to lose those bits of itself whose purpose was best served by the other.
In a Darwinian world, such dedicatedly intimate cooperation evolves only when the DNA of the parasite passes 'longitudinally' down host generations in the same vehicles as the DNA of the host. To this day, our mitochondria still have their own DNA, which is only distantly related to our 'own' DNA and more closely related to that of certain bacteria. But it passes down human generations in human eggs. Parasites whose DNA passes longitudinally like this (that is, from host parent to host child)
become less virulent and more cooperative, because anything that is
good for the host DNA's survival automatically tends to be good for their own DNA's survival. Parasites whose DNA passes 'horizontally' (from a host to some other host which is not specifically its own child), for example rabies or flu viruses, may become even more virulent. If DNA is to be transmitted horizontally, the death of the host may be no bad thing. An extreme might be a parasite that feeds inside an individual host, turning its flesh into spores until it finally bursts, scattering the parasite DNA to the winds where it is blown far and wide to find new hosts.
Mitochondria are extreme longitudinal specialists. So intimate with the host cells did they become that it is hard for us to recognize that they were ever apart. My Oxford colleague Sir David Smith has found a neat simile:
In the cell habitat, an invading organism can progressively lose pieces of itself, slowly blending into the general background, its former existence betrayed only by some relic. Indeed, one is reminded of Alice in Wonderland's encounter with the Cheshire Cat. As she watched it, "it vanished quite slowly, beginning with the tail, and ending with the grin, which remained some time after the rest of it had gone". There are a number of objects in a cell like the grin of the Cheshire Cat. For those who try to trace their origin, the grin is challenging and truly enigmatic. The Cell as a Habitat (1979)
I don't find any strong distinction between the relationship of mitochondrial DNA to host DNA and that between one gene and another within the normal orthodox gene pool of a species 'own' genes. I have argued that all our 'own' genes should be seen as mutually parasitic upon each other.
The other grinning relic which is now pretty uncontroversial is the chloroplast. Chloroplasts are small bodies in plant cells that do the business of photosynthesis - storing solar energy by using it to synthesize organic molecules. These organic molecules can then be broken down later and the energy released in a controlled way when required. Chloroplasts are responsible for the green colour of plants. It is now widely agreed that they are descended from photosynthetic bacteria, cousins of the 'blue-green' bacteria that still float free today and are responsible for 'blooms' in polluted water. The process of photosynthesis is the same in these bacteria and in (the chloroplasts of) eucaryotes. Chloroplasts, according to Margulis, were captured in a different way from mitochondria. Where the mitochondrial ancestors aggressively invaded larger hosts, the ancestors of chloroplasts were prey, originally engulfed for food, only later evolving a mutualistic rapport with their
captors, doubtless again because their DNA became transmitted longitudinally down host generations.
More controversially, Margulis believes that yet another kind of bacteria, the spirally moving spirochaetes, invaded the early eucaryotic cell and contributed such moving structures as cilia, flagella and the 'spindles' which drag the chromosomes apart in cell division. Cilia and flagella (singular, cilium and flagellum) are just different-sized versions of each other, and Margulis prefers to call both 'undulipodia'. She reserves the name flagellum for the superficially similar but actually very different whip-like structure that some bacteria use to paddle ('screw' is a more appropriate verb) themselves along. The bacterial flagellum, incidentally, is remarkable in having the only true rotating bearing in the living kingdoms. It is nature's only important example of 'the wheel', or at least the axle, before humans reinvented it. Cilia and other eucaryotic undulipodia are more complicated. Margulis identifies each individual undulipodium with an entire spirochaete bacterium, in the same way as she identifies each mitochondrion and each chloroplast with an entire bacterium.
The idea of co-opting bacteria to perform some difficult biochemical trick has frequently resurfaced in more recent evolution. Deep sea fish have luminous organs to signal to each other and even to find their way about. Rather than undertake the difficult chemical task of making light, they have co-opted bacteria that specialize in the skill. The luminous organ of a fish is a bag of carefully cultured bacteria, which give off light as a spin off from their own biochemical purposes.
So we have a whole new way of looking at an individual organism. Not only do animals and plants participate in complicated webs of interaction with each other, and with individuals of other species, in populations and communities like a tropical rainforest or a coral reef. Each individual animal or plant is a community. It is a community of billions of cells, and each one of those billions of cells is a community of thousands of bacteria. I'd go further and say that even a species' 'own' genes are a community of selfish co-operators. Now we are tempted by yet another piece of poetic science, the poetry of hierarchy. There are units within larger units, not just up to the level of the individual organism but even higher, for organisms live in communities. Is there not, at every level in the hierarchy, symbiotic cooperation between units at the next level down, units that used to be independent?
Perhaps there is some mileage in this. Termites make a very successful living out of eating wood and wood products such as books. But, yet again, the necessary chemical tricks do not come naturally to the termite's own cells. Just as the unaided eucaryote cell has to borrow the
biochemical talents of mitochondria, so termite guts, on their own, cannot digest wood. They rely on symbiotic micro-organisms to do the business of digesting the wood. The termite itself subsists on the micro- organisms and their excreta. These micro organisms are strange and specialized creatures, mostly' found nowhere else in the world but in the guts of their own species of termite. They depend on the termites (to find the wood and chew it physically into small pieces), just as the termites depend on them (to break it down to even smaller molecular pieces, using enzymes which the termites themselves can't make). Some of the micro-organisms are bacteria, some of them are protozoa (single-celled eucaryotes), and some of them are a fascinating mixture of the two. Fascinating because of a kind of evolutionary deja vu which powerfully adds plausibility to Margulis's speculation.
Mixotricha paradoxa is a flagellate protozoan which lives in the guts of the Australian termite Mastotermes darwiniensis. It has four large cilia at the front end. Margulis, of courses believes that these are themselves originally derived from symbiotic spirochaetes. But, though that may be controversial, there is a second kind of small, waving, hair-like projection about which there is no doubt. Covering the rest of the body, these look like cilia: cilia such as those that beat rhythmically to waft our eggs down our oviducts. But they are not cilia. Every one of them - and there are about half a million - is a tiny spirochaete bacterium. Indeed, there are two quite different kinds of spirochaete involved. It is these waving bacteria that propel Mixotricha around in the termite gut, and it is reported that they wave in unison. This seems hard to believe until you realize that each one could simply be provoked by its immediate neighbours.
The four large cilia at the front seem to serve only as rudders. These might be described as Mixotricha's 'own', to distinguish them from the spirochaetes that carpet the rest of the body. But of course, if Margulis is right, they are really no more Mixotricha's own than the spirochaetes: they just represent an earlier invasion. The deja vu lies in the re- enactment by new spirochaetes, of a drama that was first staged a billion years ago. As it happens, Mixotricha cannot use oxygen because there isn't enough of it in the termite gut. Otherwise, we may be sure, they'd have mitochondria inside them - relic of yet another ancient wave of bacterial invasion. But in any case they do have other symbiotic bacteria inside them which are probably performing a biochemical role rather like mitochondria, maybe assisting with the difficult task of digesting wood.
A single Mixotricha individual, therefore, is a colony containing at least half a million symbiotic bacteria of various kinds. From a functional point of view as a wood-digesting machine, a single termite is a colony of perhaps as many symbiotic micro-organisms in its gut. Don't forget that,
quite apart from the 'recent' invaders of its gut flora, a termite's 'own' cells, like the cells of any other eucaryote, are themselves colonies of much older bacteria. Finally, termites are rather special in that they themselves live in massive colonies of mostly sterile worker insects which plunder the country side more effectively than almost any other kind of animal except ants - and they are successful for the same kind of reason. Mastotermes colonies can contain up to a million individual worker termites. The species is a voracious pest in Australia, devouring telegraph poles, the plastic lining of electric cables, wooden buildings and bridges, even billiard balls. Being a colony of colonies of colonies seems to be a successful recipe for life.
I want to return to the genes' eye view and push the idea of universal symbiosis - 'living together' - to its ultimate conclusion. Margulis is rightly seen as a high priestess of symbiosis. As I said earlier, I would go even further, and regard all 'normal' nuclear genes as symbiotic in the same kind of way as mitochondrial genes. But where Margulis and Lovelock invoke the poetry of cooperation and amity as primary in the union, I want to do the opposite and regard it as a secondary consequence. At the genetic level all is selfish, but the selfish ends of genes are served by cooperation at many levels. As far as the genes themselves are concerned, the relationships among our 'own' genes are not, in principle, different from the relationship between our genes and mitochondrial genes, or our genes and those of other species. All genes are being selected for their capacity to flourish in the presence of the other genes - of whatever species - whose consequences surround them.
Collaboration within gene pools to make complex bodies is often called co-adaptation, as distinct from co-evolution. Co-adaptation usually refers to the mutual tailoring of different bits of the same kind of organism to other bits. For example, many flowers have both a bright colour to attract insects and dark lines that act as runway guides to lead insects towards the nectar. Colour, lines and nectaries assist each other. They are co- adapted to each other, the genes that make them being selected in each others presence. Co-evolution is normally used to mean mutual evolution in different species. The flowers and the insects that pollinate them evolve together - co-evolve. In this case the co-evolutionary relationship is mutually beneficial. The word co-evolution is also used for the ' hostile kind of evolving together - co-evolutionary 'arms races'.
High-speed running in predators co-evolves with high-speed running in their prey. Thick armour co-evolves with weapons and techniques for penetrating it.
Although I have just made a clear distinction between 'within species' co- adaptation and 'between species' co-evolution, we can now see that a certain amount of confusion is pardonable. If we take the view, as I have
in this chapter, that gene interactions are just gene interactions, at any level, co-adaptation turns out to be just a special case of co-evolution. As far as the genes themselves are concerned, 'within species' is not fundamentally different from 'between species'. The differences are practical. Within a species, genes meet their companions inside cells. Between species, their consequences in the outside world may meet the consequences of the other genes, out in the external world. Intermediate cases, such as intimate parasites and mitochondria, are revealing because they blur the distinction.
Sceptics of natural selection often worry along the following lines. Natural selection, they say, is a purely negative process. It weeds out the unfit. How can such a negative weeding-out play the positive role of building up complex adaptation? A large part of the answer lies in a combination of co-evolution and co-adaptation, two processes which, as we have just seen, are not so very far apart.
Co-evolution, like a human arms race, is a recipe for progressive build- up of improvements (I mean improvements in efficiency at what they do, of course; obviously, from a humane point of view, 'improvements' in armaments are just the reverse). If predators get better at their job, prey have to follow suit just to stay in the same place. And vice versa. The same goes for parasites and hosts. Escalation begets further escalation. This leads to real progressive improvement in equipment for survival, even if it does not lead to improvement in survival itself (because, after all, the other side in the arms race is improving too). So, co-evolution - arms races, the mutual evolution of genes in different gene pools - is one answer to the sceptic who thinks natural selection is a purely negative process.
The other answer is co-adaptation, the mutual evolution of genes in the same gene pool. In the cheetah gene pool, carnivorous teeth work best with carnivorous guts and carnivorous habits. Herbivorous teeth, guts and habits form an alternative complex in an antelope gene pool. At the gene level, as we have seen, selection puts together harmonious complexes, not by choosing whole complexes but by favouring each part of the complex within gene pools that are dominated by the other parts of the complex. In the shifting balance of gene pools, more than one stable solution to the same problem may exist. Once a gene pool starts to become dominated by one stable solution, further selection of selfish genes favours the ingredients of the same solution. The other solution could equally well have been favoured if the starting conditions had been different. In any case, the sceptic's worry about whether natural selection is purely a negative, subtracting process is disarmed. Natural selection is positive and constructive. It is no more negative than a sculptor subtracting marble from a block. It carves out of gene pools complexes of
mutually interacting, co-adapted genes: fundamentally selfish but pragmatically cooperating. The unit that the Darwinian sculptor carves is the gene pool of a species.
I have devoted space in the last couple of chapters to warning of bad poetry in science. But the balance of my book is the opposite. Science is poetic, ought to be poetic, has much to learn from poets and should press good poetic imagery and metaphor into its inspirational service. 'The selfish gene' is a metaphoric image, potentially a good one but capable of sadly misleading if the metaphor of personification is improperly grasped. If interpreted aright it may lead us into paths of deep understanding and fertile research. This chapter has used the metaphor of the personified gene to explain a sense in which 'selfish' genes are also 'cooperative'.
The key image to be floated in the next chapter is that of a species' genes as a detailed description of the collection of environments in which its ancestors lived - a genetic book of the dead.
10
THE GENETIC BOOK OF THE DEAD
Remember the wisdom out of the old days . . .
W. B. YEATS, The Wind Among the Reeds (1899)
The first essay I can remember writing at school was 'The Diary of a Penny'. You had to imagine yourself a coin and tell your story, of how you sat in a bank for a while until you were given out to a customer, how it felt to jangle around in his pocket with the other coins, how you were handed over to buy something, then how you were passed out as change to another customer and then . . . well, you probably wrote a similar essay yourself. It is helpful to think the same way for a gene travelling not from pocket to pocket but from body to body down the generations. And the first point that the analogy of the coin makes is that of course the personification of the gene is not to be taken literally, any more than we seven-year-olds really thought our coins could talk. Personification is sometimes a useful device, and for critics to accuse us of taking it literally is almost as stupid as taking it literally in the first place. Physicists are not literally charmed by their particles, and the critic who would so accuse them is a tiresome pedant.
The 'minting' event for a gene is the mutation that brought it into existence by altering a previous gene. Only one of many copies of the gene in the population is changed (by one mutation event, but an
identical mutation may change another copy of the gene in the gene pool at another time). The others continue to make copies of the original gene, which may now be said to be in competition with the mutant form. Making copies is of course what genes, unlike coins, are supremely good at, and our diary of a gene has to include the experiences not of the particular atoms that go to make up the DNA, but the DNA's experiences in the form of multiple copies in successive generations. As the last chapter showed, much of a gene's 'experience' of past generations consists of rubbing up against other genes of the species, and this is why they cooperate so amicably in the collective enterprise of building bodies.
Now let's ask the question whether all the genes of a species have the same ancestral 'experiences'. Mostly they do. Most buffalo genes can look back to a long line of buffalo bodies which enjoyed, or suffered, common buffalo experiences. The bodies in which these genes survived included male and female buffaloes, large and small, and so on. But there are subsets of genes with different experiences, for example the genes that determine sex. In mammals, Y chromosomes are found only in males and do not exchange genes with other chromosomes. So a gene sitting on a Y chromosome has had a limited experience of buffalo bodies: male ones only. Its experiences are largely typical of buffalo genes in general, but not entirely so. Unlike most buffalo genes it doesn't know what it is like to sit in a female buffalo. A gene that has always been on a Y chromosome since the origin of the mammals during the age of dinosaurs will have experienced male bodies of many different species, but never a female body of any kind. The case of X chromosomes is more complicated to work out. Male mammals have one X chromosome (inherited from the mother, plus one Y chromosome inherited from the father), while females have two X chromosomes (one from each parent). So each X chromosome gene has experienced both female and male bodies, but two-thirds of its experience has been in female bodies. In birds the situation is reversed. The female bird has uneven sex chromosomes (which we may as well call X and Y by analogy with mammals, although the official bird terminology is different), the male two of the same (XX). The genes on the other chromosomes have all had an equal experience of male and female bodies, but their experiences may be unequal in other respects. A gene will have spent more than its fair share of time in ancestral bodies that possess whatever qualities the gene encodes - long legs, thick horns, or whatever it may be, especially if it is a dominant gene. Almost as obviously, all genes are likely to have spent more of their ancestral time in successful than in unsuccessful bodies. There are plenty of unsuccessful bodies about and they contain their full complement of genes. But they tend not to have descendants (that is what being unsuccessful means) so, as a gene looks back through its biography of past bodies, it will observe that all of them were as a matter of fact successful (by definition), and perhaps most (but not
all) of them were equipped with what it normally takes to be successful. The difference here is that individuals who are not equipped to be successful sometimes reproduce despite their lack. And individuals who are superbly equipped to survive and reproduce under average conditions are sometimes struck by lightning.
If, like some deer, seals and monkeys, the species is one in which the males form dominance hierarchies and dominant males do most of the reproducing, it will follow that the genes of the species will have more experience of dominant male bodies than of subordinate ones. (Note that we are no longer using dominant in its technical genetic sense, whose opposite is recessive, but in its ordinary language sense, where the opposite is subordinate. ) In every- generation, most of the males are subordinate, but their genes still look back on a strong line of dominant male ancestors. In every generation, the majority are fathered by a dominant minority from the previous generation.
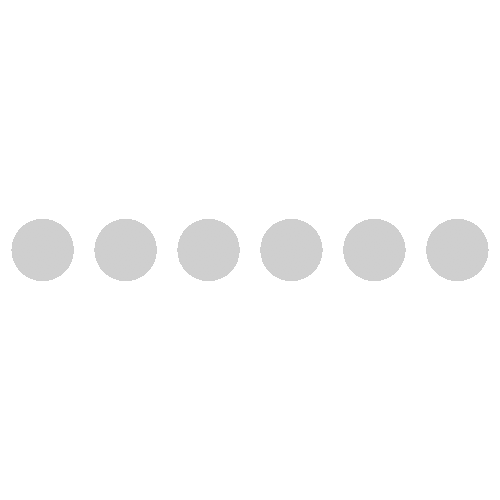