Most
demonstrations
of plasticity involve remappings within primary sensory cortex.
Steven-Pinker-The-Blank-Slate 1
Neural plasticity is just another name for learning and development, described at a different level of analysis.
All this should be obvious, but nowadays any banality about learning can be dressed up in neurospeak and treated like a great revelation of science. According to a New York Times headline, "Talk therapy, a psychiatrist maintains, can alter the structure of the patient's brain. "32 I should hope so, or else the psychiatrist would be defrauding her clients. "Environmental manipulation can change the way [a child's] brain develops," the pediatric neurologist Harry Chugani told the Boston Globe. "A child surrounded by aggression, violence, or inadequate stimulation will reflect these connections in the brain and behavior. "33 Well, yes; if the environment affects the child at all, it would do so by changing connections in the brain. A special issue of the journal Educational Technology and Society was intended "to examine the position that learning takes place in the brain of the learner, and that pedagogies and technologies
? ? ? ? ? ? ? ? ? ? ? ? should be designed and evaluated on the basis of the effect they have on student brains. " The guest editor (a
biologist) did not say whether the alternative was that learning takes place in some other organ of the body like the pancreas or that it takes place in an immaterial soul. Even professors of neuroscience sometimes proclaim "discoveries" that would be news only to believers in a ghost in the machine: "Scientists have found that the brain is capable of altering its connections. . . . . . You have the ability to change the synaptic connections within the brain. "34 Good thing, because otherwise we would be permanent amnesiacs.
This neuroscientist is an executive at a company that "uses brain research {87} and technology to develop products intended to enhance human learning and performance," one of many new companies with that aspiration. "The
human being has unlimited creativity if focused and nurtured properly," says a consultant who teaches clients to draw diagrams that "map their neural patterns. " "The older you get, the more connections and associations your brain should be making," said a satisfied customer; "Therefore you should have more information stored in your brain.
You just need to tap into it. "35 Many people have been convinced by the public pronouncements of neuroscience advocates -- on the basis of no evidence whatsoever -- that varying the route you take when driving home can stave off the effects of aging. 36 And then there is the marketing genius who realized that blocks, balls, and other toys "provide visual and tactile stimulation" and "encourage movement and tracking," part of a larger movement of "brain- based" childrearing and education that we will meet again in the chapter on children. 37
These companies tap into people's belief in a ghost in the machine by implying that any form of learning that affects the brain (as opposed, presumably, to the kinds of learning that don't affect the brain) is unexpectedly real or deep or powerful. But this is mistaken. All learning affects the brain. It is undeniably exciting when scientists make a discovery about how learning affects the brain, but that does not make the learning itself any more pervasive or profound. ~
A second misinterpretation of neural plasticity can be traced to the belief that there is nothing in the mind that was not first in the senses. The most highly publicized discoveries about cortical plasticity concern primary sensory cortex, the patches of gray matter that first receive signals from the senses (via the thalamus and other subcortical organs). Writers who use plasticity to prop up the Blank Slate assume that if primary sensory cortex is plastic, the rest of the brain must be even more plastic, because the mind is built out of sensory experience. For example, one neuroscientist was quoted as saying that Sur's rewiring experiments "challenge the recent emphasis on the power of the genes" and "will push people back toward more consideration of environmental factors in creating normal brain organization. "38 But if the brain is a complex organ with many parts, the moral does not follow. Primary sensory cortex is not the bedrock of the mind but a gadget, one of many in the brain, that happens to be specialized for certain kinds of signal processing in the first stages of sensory analysis. Let's suppose that primary sensory cortex really were formless, getting all its structure from the input. Would that mean that the entire brain is formless and gets all of its structure from the input? Not at all. For one thing, even primary sensory cortex is just one part of a huge, intricate system. To put things in perspective, here is a recent diagram of the wiring of the primate visual system:39 {88}
? ? ? ? ? ? ? ? ? Primary visual cortex is the box near the bottom labeled "V1. " It is one of at least fifty distinct brain areas devoted to visual processing, and they are interconnected in precise ways. (Despite the spaghetti-like appearance, not everything is connected to everything else. Only about a third of the logically possible connections between components are actually present in the brain. ) Primary visual cortex, by itself, is not enough to see with. Indeed, it is so deeply buried in the visual system that Francis Crick and the neuroscientist Christof Koch have argued that we are not conscious of anything that goes on in it. 40 What we see -- familiar colored objects arranged in a scene or moving in {89} particular ways -- is a product of the entire contraption. So even if the innards of the V1 box were completely specified by its input, we would have to explain the architecture of the rest of the visual system -- the fifty boxes and their connections. I don't mean to imply that the entire block diagram is genetically specified, but much of it almost certainly is. 41
And of course the visual system itself must be put into perspective, because it is just one part of the brain. The visual system dominates some half-dozen of the more than fifty major areas of the cortex that can be distinguished by their anatomy and connections. Many of the others underlie other functions such as language, reasoning, planning, and
? ? ? social skills. Though no one knows to what extent they are genetically prepared for their computational roles, there are hints that the genetic influence is substantial. 42 The divisions are established in the womb, even if the cortex is cut
off from sensory input during development. As development proceeds, different sets of genes are activated in different regions. The brain has a well-stocked toolbox of mechanisms to interconnect neurons, including molecules that attract or repel axons (the output fibers of neurons) to guide them to their targets, and molecules that glue them in place or ward them away. The number, size, and connectivity of cortical areas differ among species of mammals, and they differ between humans and other primates. This diversity is caused by genetic changes in the course of evolution that are beginning to be understood. 43 Geneticists recently discovered, for example, that different sets of genes are activated in the developing brain of humans and the developing brains of chimpanzees. 44
The possibility that cortical areas are specialized for different tasks has been obscured by the fact that different parts of the cortex look similar under a microscope. But because the brain is an information-processing system, that means little. The microscopic pits on a CD look the same regardless of what is recorded on it, and the strings of characters in different books look the same to someone who cannot read them. In an information-carrying medium, the content lies in combinatorial patterns among the elements -- in the case of the brain, the details of the microcircuitry -- and not in their physical appearance.
And the cortex itself is not the entire brain. Tucked beneath the cortex are other brain organs that drive important parts of human nature. They include the hippocampus, which consolidates memory and supports mental maps, the amygdala, which colors experience with certain emotions, and the hypothalamus, which originates sexual desire and other appetites. Many neuroscientists, even when they are impressed by the plasticity of the cortex, acknowledge that subcortical structures are far less plastic. 45 This is not a minor cavil about anatomy. Some commentators have singled out evolutionary psychology as a casualty of neural plasticity, saying that the changeability of the cortex proves that the brain cannot support evolutionary specializations. 46 But most proposals in evolutionary psychology are about drives like fear, sex, love, and {90} aggression, which reside largely in subcortical circuitry. More generally, on anyone's theory an innately shaped human ability would have to be implemented in a network of cortical and subcortical areas, not in a single patch of sensory cortex. ~
Another basic point about the brain has been lost in the recent enthusiasm for plasticity. A discovery that neural activity is crucial for brain development does not show either that learning is crucial in shaping the brain or that genes fail to shape the brain.
The study of neural development is often framed in terms of nature and nurture, but it is more fruitful to think of it as a problem in developmental biology -- how a ball of identical cells differentiates into a functioning organ. Doing so stands the conventional assumptions of associationism on their head. Primary sensory cortex, rather than being the firmest part of the brain on top of which successive stories can only be even more plastic, may be the part of the brain that is most dependent on the input for proper development.
In assembling a brain, a complete genetic blueprint is out of the question for two reasons. One is that a gene cannot anticipate every detail of the environment, including the environment consisting of the other genes in the genome. It has to specify an adaptive developmental program that ensures that the organism as a whole functions properly across variations in nutrition, other genes, growth rates over the lifespan, random perturbations, and the physical and social environment. And that requires feedback from the way the rest of the organism is developing.
Take the development of the body. The genes that build a femur cannot specify the exact shape of the ball on top, because the ball has to articulate with the socket in the pelvis, which is shaped by other genes, nutrition, age, and chance. So the ball and the socket adjust their shapes as they rotate against each other while the baby kicks in the womb. (We know this because experimental animals that are paralyzed while they develop end up with grossly deformed joints. ) Similarly, the genes shaping the lens of the growing eye cannot know how far back the retina is going to be or vice versa. So the brain of the baby is equipped with a feedback loop that uses signals about the sharpness of the image on the retina to slow down or speed up the physical growth of the eyeball. These are good examples of "plasticity," but the metaphor of plastic material is misleading. The mechanisms are not designed to allow variable environments to shape variable organs. They do the opposite: they ensure that despite variable environments, a constant organ develops, one that is capable of doing its job.
Like the body, the brain must use feedback circuits to shape itself into a working system. This is especially true in the sensory areas, which have to cope with growing sense organs. For that reason alone we would expect the activity
{91} of the brain to play a role in its own development, even if its end state, like those of the femur and the eyeball, is in some sense genetically specified. How this happens is still largely a mystery, but we know that patterns of neural stimulation can trigger the expression of a gene and that one gene can trigger many others. 47 Since every brain cell contains a complete genetic program, the machinery exists, in principle, for neural activity to trigger the development
? ? ? ? ? ? ? ? of an innately organized neural circuitry in any of several different regions. If so, brain activity would not be sculpting the brain; it would merely be telling the genome where in the brain a certain neural circuit should go.
So even an extreme innatist need not believe that the brain differentiates itself by the equivalent of GPS coordinates in the skull, following rules like "If you are between the left temple and the left ear, become a language circuit" (or a fear circuit, or a circuit for recognizing faces). A developmental program may be triggered in a part of the developing brain by some combination of the source of the stimulation, the firing pattern, the chemical environment, and other signals. The end result may be a faculty that is seated in different parts of the brain in different people. After all, the brain is the organ of computation, and the same computation can happen in different places as long as the pattern of information flow is the same. In your computer, a file or program may sit in different parts of memory or be fragmented across different sectors of the disk and work the same way in every case. It would not be surprising if the growing brain were at least that dynamic in allocating neural resources to computational demands.
The other reason that brains can't rely on a complete genetic blueprint is that the genome is a limited resource. Genes are constantly mutating over evolutionary time, and natural selection can weed out the bad ones only slowly. Most evolutionary biologists believe that natural selection can support a genome that is only so big. That means that the genetic plans for a complex brain have to be compressed to the minimum size that is consistent with the brain's developing and working properly. Though more than half the genome is put to work primarily or exclusively in the brain, that is not nearly enough to specify the brain's connection diagram.
The development program for the brain has to be resourceful. Take the problem of getting every axon (output fiber) from the eyes to connect to the brain in an orderly way. Neighboring points in the eye must connect to neighboring points in the brain (an arrangement called topographic mapping), and corresponding locations in the two eyes should end up near each other in the brain but not get mixed up with each other.
Rather than give each axon a genetically specified address, the mammalian brain may organize the connections in a cleverer way. In her studies of brain development in cats, the neuroscientist Carla Shatz has discovered that waves of activity flow across each retina, first in one direction, then in some other {92} direction. 48 That means that neurons that are next to each other in a single eye will tend to fire at around the same time, because they are often hit by the same wavefront. But axons from different eyes, or from distant locations in the same eye, will be uncorrelated in their activity, because a wave passing over one will miss the other. Just as you could reconstruct the seating diagram of a stadium if the fans were doing "the wave" along various directions and you knew only who stood up at which time (since people who stood up at the same time had to be seated near each other), the brain could reconstruct the spatial layout of the two eyes by listening for which sets of input neurons were firing at the same time. One of the rules of learning in neural networks, first outlined by the psychologist D. O. Hebb, is that "neurons that fire together wire together; neurons out of synch fail to link. " As the waves crisscross the retina for days and weeks, the visual thalamus downstream could organize itself into layers, each from a single eye, with adjacent neurons responding to adjacent parts of the retina. The cortex, in theory, could organize its wiring in a similar way. 49
Which parts of the brain actually use this auto-installation technique is another matter. The visual system does not appear to need the technique to grow topographically organized wiring; a rough topographic map develops under the direct control of the genes. Some neuroscientists believe that the fire-together-wire-together technique may still be used to make the maps more precise or to segregate the inputs from the two eyes. 50 That, too, has been challenged, but let us assume it is correct and see what it means.
The fire-together-wire-together process could, in theory, be set in motion by letting the eyeballs gaze at the world. The world has lines and edges that stimulate neighboring parts of the retina at the same time, and that provides the information the brain needs to set up or fine-tune an orderly map. But in the case of Shatz's cats, it works without any environmental input at all. The visual system develops in the pitch-dark womb, before the animal's eyes are open and before its rods and cones are even hooked up and functioning. The retinal waves are generated endogenously by the tissues of the retina during the period in which the visual brain has to wire itself up. In other words, the eye generates a test pattern, and the brain uses it to complete its own assembly. Ordinarily, axons from the eye carry information about things in the world, but the developmental program co-opted those axons to carry information about which neurons come from the same eye or the same place in the eye. A rough analogy occurred to me when I watched the cable TV installer figure out which cable in the basement led to a particular room upstairs. He attached a tone generator called a "screamer" to the end in the bedroom and then ran downstairs to listen for the signal on each cable in the bouquet coming out of the wall. Though the cables were designed to carry a television signal upstairs, not a test tone downstairs, they lent themselves to this other use during the installation process because an information conduit is useful for both purposes. {93}
The moral is that a discovery that brain development depends on brain activity may say nothing about learning or experience, only that the brain takes advantage of its own information-transmission abilities while wiring itself up. Fire-together-wire-together is a trick that solves a particular kind of wiring problem: connecting a surface of
? ? ? ? ? receptors to a maplike representation in the cortex. The problem is found not just in the visual system but in other spatial senses such as touch. That is because the problem of tiling a patch of primary visual cortex, which receives information from the 2-D surface of the retina, is similar to the problem of tiling a patch of primary somatosensory cortex, which receives information from the 2-D surface of the skin. Even the auditory system may use the trick, because the inputs representing different sound frequencies (roughly, pitches) originate in a 1-D membrane in the inner ear, and the brain treats pitch in audition the way it treats space in vision and touch.
But the trick may be useless elsewhere in the brain. The olfactory (smell) system, for example, wires itself by a completely different technique. Unlike sights, sounds, and touches, which are arranged by location when they arrive at the sensory cortex, smells arrive all mixed together, and they are analyzed in terms of the chemical compounds making them up, each detected by a different receptor in the nose. Each receptor connects to a neuron that carries its signal into the brain, and in this case the genome really does use a different gene for each axon when wiring them
into their respective places in the brain, a thousand genes in all. It economizes on genes in a remarkable way. The protein produced by each gene is used twice: once in the nose, as a receptor to detect an airborne chemical, and a second time in the brain, as a probe at the end of the corresponding axon to direct it to its proper spot in the olfactory bulb. 51
The wiring problems are different again for other parts of the brain, such as the medulla, which generates the swallowing reflex and other fixed action patterns; the amygdala, which handles fear and other emotions; and the ventromedial frontal cortex, which is involved in social reasoning. The fire-together-wire-together technique may be an ideal method for sensory maps and other structures that simply have to reproduce redundancies in the world or in other parts of the brain, such as primary sensory cortex for seeing, touching, and hearing. But other regions evolved with different functions, such as smelling or swallowing or avoiding danger or winning friends, and they have to be wired by more complicated techniques. This is simply a corollary of the general point with which I began the chapter: the environment cannot tell the various parts of an organism what their goals are.
The doctrine of extreme plasticity has used the plasticity discovered in primary sensory cortex as a metaphor for what happens elsewhere in the brain. The upshot of these two sections is that it is not a very good metaphor. If the plasticity of sensory cortex symbolized the plasticity of mental life as a whole, it should be easy to change what we don't like about ourselves or other people. {94}
Take a case very different from vision, sexual orientation. Most gay men feel stirrings of attraction to other males around the time of the first hormonal changes that presage puberty. No one knows why some boys become gay -- genes, prenatal hormones, other biological causes, and chance may all play a role -- but my point is not so much about becoming gay as about becoming straight. In the less tolerant past, unhappy gay men sometimes approached psychiatrists (and sometimes were coerced into approaching them) for help in changing their sexual orientation. Even today, some religious groups pressure their gay members to "choose" heterosexuality. Many techniques have been foisted on them: psychoanalysis, guilt mongering, and conditioning techniques that use impeccable fire-together-wire- together logic (for example, having them look at Playboy centerfolds while sexually aroused). The techniques are all failures. 52 With a few dubious exceptions (which are probably instances of conscious self-control rather than a
change in desire), the sexual orientation of most gay men cannot be reversed by experience. Some parts of the mind just aren't plastic, and no discoveries about how sensory co~rtex gets wired will change that fact.
What is the brain actually doing when it undergoes the changes we call plasticity? One commentator called it "the brain equivalent of Christ turning water into wine" and thus a disproof of any theory that parts of the brain have been specialized for their jobs by evolution. 53 Those who don't believe in miracles are skeptical. Neural tissue is not a magical substance that can assume any form demanded of it but a mechanism that obeys the laws of cause and effect. When we take a closer look at the prominent examples of plasticity, we discover that the changes are not miracles after all. In every case, the altered cortex is not doing anything very different from what it ordinarily does.
Most demonstrations of plasticity involve remappings within primary sensory cortex. A brain area for an amputated or immobilized finger may be taken over by an adjacent finger, or a brain area for a stimulated finger expands its borders at the expense of a neighbor. The brain's ability to reweight its inputs is indeed remarkable, but the kind of information processing done by the taken-over cortex has not fundamentally changed: the cortex is still processing information about the surface of the skin and the angles of the joints. And the representation of a digit or part of the visual field cannot grow indefinitely, no matter how much it is stimulated; the intrinsic wiring of the brain would prevent it. 54
What about the takeover of the visual cortex by Braille in blind people? At first glance it looks like real transubstantiation. But maybe not. We are not witnessing just any talent taking over just any vacant lot in the cortex. Braille reading may use the anatomy of the visual cortex in the same way that seeing does.
? ? ? ? ? Neuroanatomists have long known that there are as many fibers bringing {95} information down into the visual cortex from other brain areas as there are bringing information up from the eyes. 55 These top-down connections could have several uses. They may aim a spotlight of attention on portions of the visual field, or coordinate vision with the other senses, or group pixels into regions, or implement mental imagery, the ability to visualize things in the mind's eye. 56 Blind people may simply be using these prewired top-down connections to read Braille. They may be "imagining" the rows of dots as they feel them, much as a blindfolded person can imagine objects placed in his hand, though of course far more rapidly. (Previous research has established that blind people have mental images -- perhaps even visual images -- containing spatial information. )57 The visual cortex is well suited to the kind of computation needed for Braille. In sighted people the eyes scan around a scene, bringing fine detail into the fovea, the high-resolution center of the retina. This is similar to moving the hands over a line of Braille, bringing fine detail under the high-resolution skin of the fingertips. So the visual system may be functioning in blind people much as it does in sighted ones, despite the lack of input from the eyes. Years of practice at imagining the tactile world and attending to the details of Braille have led the visual cortex to make maximal use of the innate inputs from other parts of the brain.
With deafness, too, one of the senses is taking over the controls of suitable circuitry, rather than just moving into any old unoccupied territory. Laura Petitto and her colleagues found that deaf people use the superior gyrus of the temporal lobe (a region near the primary auditory cortex) to recognize the elements of signs in sign languages, just as hearing people use it to process speech sounds in spoken languages. They also found that the deaf use the lateral prefrontal cortex to retrieve signs from memory, just as hearing people use it to retrieve words from memory. 58 This should come as no surprise. As linguists have long known, sign languages are organized much like spoken languages. They use words, a grammar, and even phonological rules that combine meaningless gestures into meaningful signs, just as phonological rules in spoken languages combine meaningless sounds into meaningful words. 59 Spoken languages, moreover, are partly modular: the representations for words and rules can be distinguished from the input- output systems that connect them to the ears and the mouth. The simplest interpretation, endorsed by Petitto and her colleagues, is that the cortical areas recruited in signers are specialized for language (words and rules), not for speech per se. What the areas are doing in deaf people is the same as what they are doing in hearing people.
Let me turn to the most amazing plasticity of all: the rewired ferrets whose eyes fed their auditory thalamus and cortex and made those areas work like a visual thalamus and cortex. Even here, water is not being turned into wine. Sur and his colleagues noted the redirected input did not change the actual wiring of the auditory brain, only the pattern of synaptic strengths. As a result they {96} found many differences between the co-opted auditory brain and a normal visual brain. 60 The representation of the visual field in the auditory brain was fuzzier and more disorganized, because the tissue is optimized for auditory, not visual, analysis. The map of the visual field, for instance, was far more precise in the left-right direction than in the up-down direction. That is because the left-right direction was mapped onto an axis of the auditory cortex that in normal animals represents different sound frequencies and thus gets inputs from the inner ear that are precisely arranged in order of frequency. But the up-down direction was mapped onto the perpendicular axis of the auditory cortex, which ordinarily gets a mass of inputs of the same frequency. Sur also notes that the connections between the primary auditory cortex and other brain areas for hearing (the equivalent of the wiring diagram for the visual system on page 88) were unchanged by the new input.
So patterns in the input can tune a patch of sensory cortex to mesh with that input, but only within the limits of the wiring already present. Sur suggests that the reason the auditory cortex in the rewired ferrets can process visual information at all is that certain kinds of signal processing may be useful to perform on raw sensory input, whether it is visual, auditory, or tactile:
On this view, one function of sensory thalamus or cortex is to perform certain stereotypical operations on input regardless of modality [vision, hearing, or touch]; the specific type of sensory input of course provides the substrate information that is transmitted and processed. . . . If the normal organization of central auditory structures is not altered, or at least not altered significantly, by visual input, then we might expect some operations similar to those we observe on visual inputs in operated ferrets to be carried out as well in the auditory pathway in normal ferrets. In other words, the animals with visual inputs induced into the auditory pathway provide a different window on some of the same operations that should occur normally in auditory thalamus and cortex. 61
The suggestion that the auditory cortex is inherently suited to analyze visual input is not far-fetched. I mentioned that frequency (pitch) in hearing behaves a lot like space in vision. The mind treats soundmakers with different pitches as if they were objects at different locations, and it treats jumps in pitch like motions in space. 62 This means that some of the analyses performed on sights may be the same as the analyses performed on sounds, and could be computed, at
? ? ? ? ? ? ? ? ? ? least in part, by similar kinds of circuitry. Inputs from an ear represent different frequencies; inputs from an eye represent spots at different locations. Neurons in the sensory cortex (both visual and auditory) receive information from a neighborhood of input fibers and extract simple patterns from them. Therefore neurons in the auditory cortex that ordinarily detect {97} rising or falling glides, rich or pure tones, and sounds that come from specific places may, in the rewired ferrets, automatically be capable of detecting lines of specific slants, places, and directions of movement.
This is not to say that the primary auditory cortex can handle visual input right out of the box. The cortex still must tune its synaptic connections in response to the patterns in the input. The rewired ferrets are a remarkable demonstration of how the developing sensory cortex organizes itself into a well-functioning system. But as in the other examples of plasticity, they do not show that input from the senses can transform an amorphous brain into doing whatever would come in handy. The cortex has an intrinsic structure that allows it to perform certain kinds of computation. Many examples of "plasticity" may consist o~f making the input mesh with that structure.
Anyone who has watched the Discovery Channel has seen footage of baby wildebeests or zebras falling out of the birth canal, wobbling on shaky legs for a minute or two, and then prancing around their mothers with their senses, drives, and motor control fully operational. It happens far too quickly for patterned experience to have organized their brains, so there must be genetic mechanisms capable of shaping the brain before birth. Neuroscientists were aware of this before plasticity came into vogue. The first studies of the development of the visual system by David Hubel and Torsten Wiesel showed that the microcircuitry of monkeys is pretty much complete at birth. 63 Even their famous demonstrations that the visual systems of cats can be altered by experience during a critical period of development (by being reared in the dark, in striped cylinders, or with one eye sewn shut) show only that experience is necessary to maintain the visual system and to retune it as the animal grows. They do not show that experience is necessary to wire up the brain to start with.
We know in a general way how the brain assembles itself under the guidance of the genes. 64 Even before the cortex has been formed, the neurons destined to make up different areas are organized into a"proto-map. " Each area in the proto-map is composed of neurons with different properties, molecular mechanisms that attract different input fibers, and different patterns of responses to the input. Axons are attracted and repelled by many kinds of molecules dissolved in the surrounding fluid or attached to the membranes of neighboring cells. And different sets of genes are expressed in different parts of the growing cortex. The neuroscientist Lawrence Katz has lamented that fire-together- wire-together has become a "dogma" keeping neuroscientists from exploring the full reach of these genetic mechanisms. 65
But the tide is beginning to turn, and recent discoveries are showing how parts of the brain can organize themselves without any information from the senses. In experiments that the journal Science called "heretical," Katz's team removed one or both eyes from a developing ferret, depriving the visual cortex {98} of all its input. Nonetheless, the visual cortex developed with the standard arrangement of connections from the two eyes. 66
Genetically engineered mice have provided especially important clues, because knocking out a single gene can be more precise than the conventional techniques of poisoning neurons or slicing up the brain. One team invented a mouse whose synapses were completely shut down, preventing neurons from signaling to one another. Its brain developed fairly normally, complete with layered structures, fiber pathways, and synapses in the right places. 67 (The brain degenerated quickly after birth, showing again that neural activity may be more important in maintaining the brain than in wiring it. ) Another team designed a mouse with a useless thalamus, depriving the entire cortex of its input. But the cortex differentiated into the normal layers and regions, each with a different set of turned-on genes. 68 A third study did the opposite, inventing mice that were missing one of the genes that lay down gradients of molecules that help organize the brain by triggering other genes in particular places. The missing gene made a big difference: the boundaries among cortical areas were badly warped. 69 The studies with knockout mice, then, suggest that genes may be more important than neural activity in organizing the cortex. Neural activity undoubtedly plays a role, which depends on the species, the stage of development, and the part of the brain, but it is just one capability of the brain rather than the source of its structure.
What about our own species? Recall that a recent study of twins showed that differences in the anatomy of the cortex, particularly the amount of gray matter in different cortical regions, are under genetic control, paralleling differences in intelligence and other psychological traits. 70 And demonstrations of the plasticity of the human brain do not rule out substantial genetic organization. One of the most commonly cited examples of plasticity in both humans and monkeys is that the cortex dedicated to an amputated or numbed body part may get reallocated to some other body part. But the fact that the input can change the brain once it is built does not mean that the input molded the brain in the first place. Most amputees experience phantom limbs: vivid, detailed hallucinations of the missing body part.
? ? ? ? ? ? ? ? ? ? Amazingly, a substantial proportion of people who were born with a limb missing experience these apparitions as well. 71 They can describe the anatomy of their phantom limb (for example, how many toes they feel in a nonexistent
foot) and may even feel that they are gesturing with their phantom hands during conversation. One girl solved arithmetic problems by counting on her phantom fingers! The psychologist Ronald Melzack, who documented many of these cases, proposed that the brain contains an innate "neuromatrix," distributed across several cortical and subcortical regions, dedicated to representing the body.
The impression that human brains are limitlessly plastic has also come from demonstrations that children can sometimes recover from early brain {99} damage. But the existence of cerebral palsy -- lifelong difficulties with motor control and speech caused by malformations or early damage in the brain -- shows that even the plasticity of a child's brain has severe limits. The most famous evidence for extreme plasticity in humans had been the ability of some children to grow up relatively normal even with an entire hemisphere surgically removed in infancy. 72 But that may be a special case, which arises from the fact that the primate brain is fundamentally a symmetrical organ. The typically human asymmetries -- language more on the left, spatial attention and some emotions more on the right -- are superimposed on that mostly symmetrical design. It would not be surprising if the hemispheres were genetically programmed with pretty much the same abilities, together with small biases that lead each hemisphere to specialize in some talents while letting others wither. With one hemisphere gone, the remaining one has to put all its capabilities to full use.
What happens when a child loses a part of the cortex in both hemispheres, so neither hemisphere can take over the job of the missing part in the other? If cortical regions are interchangeable, plastic, and organized by the input, then an intact part of the brain should take over the function of the missing parts. The child may be a bit slower because he is working with less brain tissue, but he should develop a full complement of human faculties. But that is not what seems to happen. Several decades ago, neurologists studied a boy who suffered a temporary loss of oxygen to the brain and lost both the standard language areas in the left hemisphere and their mirror images on the right. Though he was just ten days old when he sustained the damage, he grew into a child with permanent difficulties in speaking and understanding. 73
That case study, like many in pediatric neurology, is not scientifically pure, but recent studies on two other mental faculties echo the point that babies' brains may be less plastic than many people think. The psychologist Martha Farah and her collaborators recently reported the case of a sixteen-year-old boy who contracted meningitis when he was one day old and suffered damage to the visual cortex and to the bottom of the temporal lobes on both sides of his brain. 74 When adults sustain such damage, they lose the ability to recognize faces and also have some trouble recognizing animals, though they often can recognize words, tools, furniture, and other shapes. The boy had exactly this syndrome. Though he grew up with normal verbal intelligence, he was utterly incapable of recognizing faces. He could not even recognize pictures of the cast of his favorite television show, Baywatch, which he had seen for an hour a day for the preceding year and a half. Without the appropriate strips of brain, sixteen years of seeing faces and plenty of available cortex were not enough to give him the basic human ability to recognize other people by sight. The neuroscientists Steven Anderson, Hannah and Antonio Damasio, and their colleagues recently tested two young adults who had sustained damage to {100} their ventromedial and orbital prefrontal cortex when they were young children. 75 These are the parts of the brain that sit above the eyes and are important for empathy, social skills, and self-management (as we know from Phineas Gage, the railroad worker whose brain was impaled by a tamping iron). Both children recovered from their injuries and grew up with average IQs in stable homes with normal siblings and college-educated parents. If the brain were really homogeneous and plastic, the healthy parts should have been shaped by the normal social environment and taken oyer the functions of the damaged parts. But that is not what happened with either of the children. One, who had been run over by a car when she was fifteen months old, grew into an intractable child who ignored punishment and lied compulsively. As a teenager she shoplifted, stole from her parents, failed to win friends, showed no empathy or remorse, and was dangerously uninterested in her own baby. The other patient was a young man who had lost similar parts of his brain to a tumor when he was three months old. He too grew up friendless, shiftless, thieving, and hotheaded. Along with their bad behavior, both had trouble thinking through simple moral problems, despite having IQs in the normal range. They could not, for example, say what two people should do if they disagreed on which TV channel to watch, or decide whether a man ought to steal a drug to save his dying wife.
These cases do more than refute the doctrine of extreme plasticity. They set a challenge for the genetics and neuroscience of the twenty-first century. How does the genome tell a developing brain to differentiate into neural networks that are prepared for such abstract computational problems as recognizing a face or thinking about the interests of other people? ~
? ? ? ? ? ? ? The Blank Slate has made its last stand, but, as we have seen, its latest scientific fortifications are illusory. The human genome may have a smaller number of genes than biologists had previously estimated, but that only shows that the number of genes in a genome has little to do with the complexity of the organism. Connectionist networks may explain some of the building blocks of cognition, but they are too underpowered to account for thought and language on their own; they must be innately engineered and assembled for the tasks. Neural plasticity is not a magical protean power of the brain but a set of tools that help turn megabytes of genome into terabytes of brain, that make sensory cortex dovetail with its input, and that implement the process called learning.
Therefore genomics, neural networks, and neural plasticity fit into the picture that has emerged in recent decades of a complex human nature. It is not, of course, a nature that is rigidly programmed, impervious to the input, free of culture, or endowed with the minutiae of every concept and feeling. But it is a nature that is rich enough to take on the demands of seeing, moving, {101} planning, talking, staying alive, making sense of the environment, and negotiating the world of other people.
The aftermath of the Blank Slate's last stand is a good time to take stock of the case for the alternative. Here is my summary of the evidence for a complex human nature, some of it reiterating arguments from previous chapters, some of it anticipating arguments in chapters to come.
Simple logic says there can be no learning without innate mechanisms to do the learning. Those mechanisms must be powerful enough to account for all the kinds of learning that humans accomplish. Learnability theory -- the mathematical analysis of how learning can work in principle -- tells us there are always an infinite number of generalizations that a learner can draw from a finite set of inputs. 76 The sentences heard by a child, for example, can be grounds for repeating them back verbatim, producing any combination of words with the same proportion of nouns to verbs, or analyzing the underlying grammar and producing sentences that conform to it. The sight of someone washing dishes can, with equal logical justification, prompt a learner to try to get dishes clean or to let warm water run over his fingers. A successful learner, then, must be constrained to draw some conclusions from the input and not others. Artificial intelligence reinforces this point. Computers and robots programmed to do humanlike feats are invariably endowed with many complex modules. 77
Evolutionary biology has shown that complex adaptations are ubiquitous in the living world, and that natural selection is capable of evolving them, including complex cognitive and behavioral adaptations. 78 The study of the behavior of animals in their natural habitat shows that species differ innately from one another in their drives and abilities, some of them (like celestial navigation and food caching) requiring complicated and specialized neural systems. 79 The study of humans from an evolutionary perspective has shown that many psychological faculties (such as our hunger for fatty food, for social status, and for risky sexual liaisons) are better adapted to the evolutionary demands of our ancestral environment than to the actual demands of the current environment. 80 Anthropological surveys have shown that hundreds of universals, pertaining to every aspect of experience, cut across the world's cultures. 81
Cognitive scientists have discovered that distinct kinds of representations and processes are used in different domains of knowledge, such as words and rules for language, the concept of an enduring object for understanding the physical world, and a theory of mind for understanding other people. 82 Developmental psychology has shown that these distinct modes of interpreting experience come on line early in life: infants have a basic grasp of objects, numbers, faces, tools, language, and other domains of human cognition. 83
The human genome contains an enormous amount of information, both in the genes and in the noncoding regions, to guide the construction of a {102} complex organism. In a growing number of cases, particular genes can be tied to aspects of cognition, language, and personality. 84 When psychological traits vary, much of the variation comes from differences in genes: identical twins are more similar than fraternal twins, and biological siblings are more similar than adoptive siblings, whether reared together or apart. 85 A person's temperament and personality emerge early in life and remain fairly constant throughout the lifespan. 86 And both personality and intelligence show few or no effects of children's particular home environments within their culture: children reared in the same family are similar mainly because of their shared genes. 87
Finally, neuroscience is showing that the brain's basic architecture develops under genetic control. The importance of learning and plasticity notwithstanding, brain systems show signs of innate specialization and cannot arbitrarily substitute for one another. 88
In these three chapters I have given you a summary of the current scientific case for a complex human nature. The rest of the book is about its implications.
? ? ? ? ? ? ? ? ? ?
All this should be obvious, but nowadays any banality about learning can be dressed up in neurospeak and treated like a great revelation of science. According to a New York Times headline, "Talk therapy, a psychiatrist maintains, can alter the structure of the patient's brain. "32 I should hope so, or else the psychiatrist would be defrauding her clients. "Environmental manipulation can change the way [a child's] brain develops," the pediatric neurologist Harry Chugani told the Boston Globe. "A child surrounded by aggression, violence, or inadequate stimulation will reflect these connections in the brain and behavior. "33 Well, yes; if the environment affects the child at all, it would do so by changing connections in the brain. A special issue of the journal Educational Technology and Society was intended "to examine the position that learning takes place in the brain of the learner, and that pedagogies and technologies
? ? ? ? ? ? ? ? ? ? ? ? should be designed and evaluated on the basis of the effect they have on student brains. " The guest editor (a
biologist) did not say whether the alternative was that learning takes place in some other organ of the body like the pancreas or that it takes place in an immaterial soul. Even professors of neuroscience sometimes proclaim "discoveries" that would be news only to believers in a ghost in the machine: "Scientists have found that the brain is capable of altering its connections. . . . . . You have the ability to change the synaptic connections within the brain. "34 Good thing, because otherwise we would be permanent amnesiacs.
This neuroscientist is an executive at a company that "uses brain research {87} and technology to develop products intended to enhance human learning and performance," one of many new companies with that aspiration. "The
human being has unlimited creativity if focused and nurtured properly," says a consultant who teaches clients to draw diagrams that "map their neural patterns. " "The older you get, the more connections and associations your brain should be making," said a satisfied customer; "Therefore you should have more information stored in your brain.
You just need to tap into it. "35 Many people have been convinced by the public pronouncements of neuroscience advocates -- on the basis of no evidence whatsoever -- that varying the route you take when driving home can stave off the effects of aging. 36 And then there is the marketing genius who realized that blocks, balls, and other toys "provide visual and tactile stimulation" and "encourage movement and tracking," part of a larger movement of "brain- based" childrearing and education that we will meet again in the chapter on children. 37
These companies tap into people's belief in a ghost in the machine by implying that any form of learning that affects the brain (as opposed, presumably, to the kinds of learning that don't affect the brain) is unexpectedly real or deep or powerful. But this is mistaken. All learning affects the brain. It is undeniably exciting when scientists make a discovery about how learning affects the brain, but that does not make the learning itself any more pervasive or profound. ~
A second misinterpretation of neural plasticity can be traced to the belief that there is nothing in the mind that was not first in the senses. The most highly publicized discoveries about cortical plasticity concern primary sensory cortex, the patches of gray matter that first receive signals from the senses (via the thalamus and other subcortical organs). Writers who use plasticity to prop up the Blank Slate assume that if primary sensory cortex is plastic, the rest of the brain must be even more plastic, because the mind is built out of sensory experience. For example, one neuroscientist was quoted as saying that Sur's rewiring experiments "challenge the recent emphasis on the power of the genes" and "will push people back toward more consideration of environmental factors in creating normal brain organization. "38 But if the brain is a complex organ with many parts, the moral does not follow. Primary sensory cortex is not the bedrock of the mind but a gadget, one of many in the brain, that happens to be specialized for certain kinds of signal processing in the first stages of sensory analysis. Let's suppose that primary sensory cortex really were formless, getting all its structure from the input. Would that mean that the entire brain is formless and gets all of its structure from the input? Not at all. For one thing, even primary sensory cortex is just one part of a huge, intricate system. To put things in perspective, here is a recent diagram of the wiring of the primate visual system:39 {88}
? ? ? ? ? ? ? ? ? Primary visual cortex is the box near the bottom labeled "V1. " It is one of at least fifty distinct brain areas devoted to visual processing, and they are interconnected in precise ways. (Despite the spaghetti-like appearance, not everything is connected to everything else. Only about a third of the logically possible connections between components are actually present in the brain. ) Primary visual cortex, by itself, is not enough to see with. Indeed, it is so deeply buried in the visual system that Francis Crick and the neuroscientist Christof Koch have argued that we are not conscious of anything that goes on in it. 40 What we see -- familiar colored objects arranged in a scene or moving in {89} particular ways -- is a product of the entire contraption. So even if the innards of the V1 box were completely specified by its input, we would have to explain the architecture of the rest of the visual system -- the fifty boxes and their connections. I don't mean to imply that the entire block diagram is genetically specified, but much of it almost certainly is. 41
And of course the visual system itself must be put into perspective, because it is just one part of the brain. The visual system dominates some half-dozen of the more than fifty major areas of the cortex that can be distinguished by their anatomy and connections. Many of the others underlie other functions such as language, reasoning, planning, and
? ? ? social skills. Though no one knows to what extent they are genetically prepared for their computational roles, there are hints that the genetic influence is substantial. 42 The divisions are established in the womb, even if the cortex is cut
off from sensory input during development. As development proceeds, different sets of genes are activated in different regions. The brain has a well-stocked toolbox of mechanisms to interconnect neurons, including molecules that attract or repel axons (the output fibers of neurons) to guide them to their targets, and molecules that glue them in place or ward them away. The number, size, and connectivity of cortical areas differ among species of mammals, and they differ between humans and other primates. This diversity is caused by genetic changes in the course of evolution that are beginning to be understood. 43 Geneticists recently discovered, for example, that different sets of genes are activated in the developing brain of humans and the developing brains of chimpanzees. 44
The possibility that cortical areas are specialized for different tasks has been obscured by the fact that different parts of the cortex look similar under a microscope. But because the brain is an information-processing system, that means little. The microscopic pits on a CD look the same regardless of what is recorded on it, and the strings of characters in different books look the same to someone who cannot read them. In an information-carrying medium, the content lies in combinatorial patterns among the elements -- in the case of the brain, the details of the microcircuitry -- and not in their physical appearance.
And the cortex itself is not the entire brain. Tucked beneath the cortex are other brain organs that drive important parts of human nature. They include the hippocampus, which consolidates memory and supports mental maps, the amygdala, which colors experience with certain emotions, and the hypothalamus, which originates sexual desire and other appetites. Many neuroscientists, even when they are impressed by the plasticity of the cortex, acknowledge that subcortical structures are far less plastic. 45 This is not a minor cavil about anatomy. Some commentators have singled out evolutionary psychology as a casualty of neural plasticity, saying that the changeability of the cortex proves that the brain cannot support evolutionary specializations. 46 But most proposals in evolutionary psychology are about drives like fear, sex, love, and {90} aggression, which reside largely in subcortical circuitry. More generally, on anyone's theory an innately shaped human ability would have to be implemented in a network of cortical and subcortical areas, not in a single patch of sensory cortex. ~
Another basic point about the brain has been lost in the recent enthusiasm for plasticity. A discovery that neural activity is crucial for brain development does not show either that learning is crucial in shaping the brain or that genes fail to shape the brain.
The study of neural development is often framed in terms of nature and nurture, but it is more fruitful to think of it as a problem in developmental biology -- how a ball of identical cells differentiates into a functioning organ. Doing so stands the conventional assumptions of associationism on their head. Primary sensory cortex, rather than being the firmest part of the brain on top of which successive stories can only be even more plastic, may be the part of the brain that is most dependent on the input for proper development.
In assembling a brain, a complete genetic blueprint is out of the question for two reasons. One is that a gene cannot anticipate every detail of the environment, including the environment consisting of the other genes in the genome. It has to specify an adaptive developmental program that ensures that the organism as a whole functions properly across variations in nutrition, other genes, growth rates over the lifespan, random perturbations, and the physical and social environment. And that requires feedback from the way the rest of the organism is developing.
Take the development of the body. The genes that build a femur cannot specify the exact shape of the ball on top, because the ball has to articulate with the socket in the pelvis, which is shaped by other genes, nutrition, age, and chance. So the ball and the socket adjust their shapes as they rotate against each other while the baby kicks in the womb. (We know this because experimental animals that are paralyzed while they develop end up with grossly deformed joints. ) Similarly, the genes shaping the lens of the growing eye cannot know how far back the retina is going to be or vice versa. So the brain of the baby is equipped with a feedback loop that uses signals about the sharpness of the image on the retina to slow down or speed up the physical growth of the eyeball. These are good examples of "plasticity," but the metaphor of plastic material is misleading. The mechanisms are not designed to allow variable environments to shape variable organs. They do the opposite: they ensure that despite variable environments, a constant organ develops, one that is capable of doing its job.
Like the body, the brain must use feedback circuits to shape itself into a working system. This is especially true in the sensory areas, which have to cope with growing sense organs. For that reason alone we would expect the activity
{91} of the brain to play a role in its own development, even if its end state, like those of the femur and the eyeball, is in some sense genetically specified. How this happens is still largely a mystery, but we know that patterns of neural stimulation can trigger the expression of a gene and that one gene can trigger many others. 47 Since every brain cell contains a complete genetic program, the machinery exists, in principle, for neural activity to trigger the development
? ? ? ? ? ? ? ? of an innately organized neural circuitry in any of several different regions. If so, brain activity would not be sculpting the brain; it would merely be telling the genome where in the brain a certain neural circuit should go.
So even an extreme innatist need not believe that the brain differentiates itself by the equivalent of GPS coordinates in the skull, following rules like "If you are between the left temple and the left ear, become a language circuit" (or a fear circuit, or a circuit for recognizing faces). A developmental program may be triggered in a part of the developing brain by some combination of the source of the stimulation, the firing pattern, the chemical environment, and other signals. The end result may be a faculty that is seated in different parts of the brain in different people. After all, the brain is the organ of computation, and the same computation can happen in different places as long as the pattern of information flow is the same. In your computer, a file or program may sit in different parts of memory or be fragmented across different sectors of the disk and work the same way in every case. It would not be surprising if the growing brain were at least that dynamic in allocating neural resources to computational demands.
The other reason that brains can't rely on a complete genetic blueprint is that the genome is a limited resource. Genes are constantly mutating over evolutionary time, and natural selection can weed out the bad ones only slowly. Most evolutionary biologists believe that natural selection can support a genome that is only so big. That means that the genetic plans for a complex brain have to be compressed to the minimum size that is consistent with the brain's developing and working properly. Though more than half the genome is put to work primarily or exclusively in the brain, that is not nearly enough to specify the brain's connection diagram.
The development program for the brain has to be resourceful. Take the problem of getting every axon (output fiber) from the eyes to connect to the brain in an orderly way. Neighboring points in the eye must connect to neighboring points in the brain (an arrangement called topographic mapping), and corresponding locations in the two eyes should end up near each other in the brain but not get mixed up with each other.
Rather than give each axon a genetically specified address, the mammalian brain may organize the connections in a cleverer way. In her studies of brain development in cats, the neuroscientist Carla Shatz has discovered that waves of activity flow across each retina, first in one direction, then in some other {92} direction. 48 That means that neurons that are next to each other in a single eye will tend to fire at around the same time, because they are often hit by the same wavefront. But axons from different eyes, or from distant locations in the same eye, will be uncorrelated in their activity, because a wave passing over one will miss the other. Just as you could reconstruct the seating diagram of a stadium if the fans were doing "the wave" along various directions and you knew only who stood up at which time (since people who stood up at the same time had to be seated near each other), the brain could reconstruct the spatial layout of the two eyes by listening for which sets of input neurons were firing at the same time. One of the rules of learning in neural networks, first outlined by the psychologist D. O. Hebb, is that "neurons that fire together wire together; neurons out of synch fail to link. " As the waves crisscross the retina for days and weeks, the visual thalamus downstream could organize itself into layers, each from a single eye, with adjacent neurons responding to adjacent parts of the retina. The cortex, in theory, could organize its wiring in a similar way. 49
Which parts of the brain actually use this auto-installation technique is another matter. The visual system does not appear to need the technique to grow topographically organized wiring; a rough topographic map develops under the direct control of the genes. Some neuroscientists believe that the fire-together-wire-together technique may still be used to make the maps more precise or to segregate the inputs from the two eyes. 50 That, too, has been challenged, but let us assume it is correct and see what it means.
The fire-together-wire-together process could, in theory, be set in motion by letting the eyeballs gaze at the world. The world has lines and edges that stimulate neighboring parts of the retina at the same time, and that provides the information the brain needs to set up or fine-tune an orderly map. But in the case of Shatz's cats, it works without any environmental input at all. The visual system develops in the pitch-dark womb, before the animal's eyes are open and before its rods and cones are even hooked up and functioning. The retinal waves are generated endogenously by the tissues of the retina during the period in which the visual brain has to wire itself up. In other words, the eye generates a test pattern, and the brain uses it to complete its own assembly. Ordinarily, axons from the eye carry information about things in the world, but the developmental program co-opted those axons to carry information about which neurons come from the same eye or the same place in the eye. A rough analogy occurred to me when I watched the cable TV installer figure out which cable in the basement led to a particular room upstairs. He attached a tone generator called a "screamer" to the end in the bedroom and then ran downstairs to listen for the signal on each cable in the bouquet coming out of the wall. Though the cables were designed to carry a television signal upstairs, not a test tone downstairs, they lent themselves to this other use during the installation process because an information conduit is useful for both purposes. {93}
The moral is that a discovery that brain development depends on brain activity may say nothing about learning or experience, only that the brain takes advantage of its own information-transmission abilities while wiring itself up. Fire-together-wire-together is a trick that solves a particular kind of wiring problem: connecting a surface of
? ? ? ? ? receptors to a maplike representation in the cortex. The problem is found not just in the visual system but in other spatial senses such as touch. That is because the problem of tiling a patch of primary visual cortex, which receives information from the 2-D surface of the retina, is similar to the problem of tiling a patch of primary somatosensory cortex, which receives information from the 2-D surface of the skin. Even the auditory system may use the trick, because the inputs representing different sound frequencies (roughly, pitches) originate in a 1-D membrane in the inner ear, and the brain treats pitch in audition the way it treats space in vision and touch.
But the trick may be useless elsewhere in the brain. The olfactory (smell) system, for example, wires itself by a completely different technique. Unlike sights, sounds, and touches, which are arranged by location when they arrive at the sensory cortex, smells arrive all mixed together, and they are analyzed in terms of the chemical compounds making them up, each detected by a different receptor in the nose. Each receptor connects to a neuron that carries its signal into the brain, and in this case the genome really does use a different gene for each axon when wiring them
into their respective places in the brain, a thousand genes in all. It economizes on genes in a remarkable way. The protein produced by each gene is used twice: once in the nose, as a receptor to detect an airborne chemical, and a second time in the brain, as a probe at the end of the corresponding axon to direct it to its proper spot in the olfactory bulb. 51
The wiring problems are different again for other parts of the brain, such as the medulla, which generates the swallowing reflex and other fixed action patterns; the amygdala, which handles fear and other emotions; and the ventromedial frontal cortex, which is involved in social reasoning. The fire-together-wire-together technique may be an ideal method for sensory maps and other structures that simply have to reproduce redundancies in the world or in other parts of the brain, such as primary sensory cortex for seeing, touching, and hearing. But other regions evolved with different functions, such as smelling or swallowing or avoiding danger or winning friends, and they have to be wired by more complicated techniques. This is simply a corollary of the general point with which I began the chapter: the environment cannot tell the various parts of an organism what their goals are.
The doctrine of extreme plasticity has used the plasticity discovered in primary sensory cortex as a metaphor for what happens elsewhere in the brain. The upshot of these two sections is that it is not a very good metaphor. If the plasticity of sensory cortex symbolized the plasticity of mental life as a whole, it should be easy to change what we don't like about ourselves or other people. {94}
Take a case very different from vision, sexual orientation. Most gay men feel stirrings of attraction to other males around the time of the first hormonal changes that presage puberty. No one knows why some boys become gay -- genes, prenatal hormones, other biological causes, and chance may all play a role -- but my point is not so much about becoming gay as about becoming straight. In the less tolerant past, unhappy gay men sometimes approached psychiatrists (and sometimes were coerced into approaching them) for help in changing their sexual orientation. Even today, some religious groups pressure their gay members to "choose" heterosexuality. Many techniques have been foisted on them: psychoanalysis, guilt mongering, and conditioning techniques that use impeccable fire-together-wire- together logic (for example, having them look at Playboy centerfolds while sexually aroused). The techniques are all failures. 52 With a few dubious exceptions (which are probably instances of conscious self-control rather than a
change in desire), the sexual orientation of most gay men cannot be reversed by experience. Some parts of the mind just aren't plastic, and no discoveries about how sensory co~rtex gets wired will change that fact.
What is the brain actually doing when it undergoes the changes we call plasticity? One commentator called it "the brain equivalent of Christ turning water into wine" and thus a disproof of any theory that parts of the brain have been specialized for their jobs by evolution. 53 Those who don't believe in miracles are skeptical. Neural tissue is not a magical substance that can assume any form demanded of it but a mechanism that obeys the laws of cause and effect. When we take a closer look at the prominent examples of plasticity, we discover that the changes are not miracles after all. In every case, the altered cortex is not doing anything very different from what it ordinarily does.
Most demonstrations of plasticity involve remappings within primary sensory cortex. A brain area for an amputated or immobilized finger may be taken over by an adjacent finger, or a brain area for a stimulated finger expands its borders at the expense of a neighbor. The brain's ability to reweight its inputs is indeed remarkable, but the kind of information processing done by the taken-over cortex has not fundamentally changed: the cortex is still processing information about the surface of the skin and the angles of the joints. And the representation of a digit or part of the visual field cannot grow indefinitely, no matter how much it is stimulated; the intrinsic wiring of the brain would prevent it. 54
What about the takeover of the visual cortex by Braille in blind people? At first glance it looks like real transubstantiation. But maybe not. We are not witnessing just any talent taking over just any vacant lot in the cortex. Braille reading may use the anatomy of the visual cortex in the same way that seeing does.
? ? ? ? ? Neuroanatomists have long known that there are as many fibers bringing {95} information down into the visual cortex from other brain areas as there are bringing information up from the eyes. 55 These top-down connections could have several uses. They may aim a spotlight of attention on portions of the visual field, or coordinate vision with the other senses, or group pixels into regions, or implement mental imagery, the ability to visualize things in the mind's eye. 56 Blind people may simply be using these prewired top-down connections to read Braille. They may be "imagining" the rows of dots as they feel them, much as a blindfolded person can imagine objects placed in his hand, though of course far more rapidly. (Previous research has established that blind people have mental images -- perhaps even visual images -- containing spatial information. )57 The visual cortex is well suited to the kind of computation needed for Braille. In sighted people the eyes scan around a scene, bringing fine detail into the fovea, the high-resolution center of the retina. This is similar to moving the hands over a line of Braille, bringing fine detail under the high-resolution skin of the fingertips. So the visual system may be functioning in blind people much as it does in sighted ones, despite the lack of input from the eyes. Years of practice at imagining the tactile world and attending to the details of Braille have led the visual cortex to make maximal use of the innate inputs from other parts of the brain.
With deafness, too, one of the senses is taking over the controls of suitable circuitry, rather than just moving into any old unoccupied territory. Laura Petitto and her colleagues found that deaf people use the superior gyrus of the temporal lobe (a region near the primary auditory cortex) to recognize the elements of signs in sign languages, just as hearing people use it to process speech sounds in spoken languages. They also found that the deaf use the lateral prefrontal cortex to retrieve signs from memory, just as hearing people use it to retrieve words from memory. 58 This should come as no surprise. As linguists have long known, sign languages are organized much like spoken languages. They use words, a grammar, and even phonological rules that combine meaningless gestures into meaningful signs, just as phonological rules in spoken languages combine meaningless sounds into meaningful words. 59 Spoken languages, moreover, are partly modular: the representations for words and rules can be distinguished from the input- output systems that connect them to the ears and the mouth. The simplest interpretation, endorsed by Petitto and her colleagues, is that the cortical areas recruited in signers are specialized for language (words and rules), not for speech per se. What the areas are doing in deaf people is the same as what they are doing in hearing people.
Let me turn to the most amazing plasticity of all: the rewired ferrets whose eyes fed their auditory thalamus and cortex and made those areas work like a visual thalamus and cortex. Even here, water is not being turned into wine. Sur and his colleagues noted the redirected input did not change the actual wiring of the auditory brain, only the pattern of synaptic strengths. As a result they {96} found many differences between the co-opted auditory brain and a normal visual brain. 60 The representation of the visual field in the auditory brain was fuzzier and more disorganized, because the tissue is optimized for auditory, not visual, analysis. The map of the visual field, for instance, was far more precise in the left-right direction than in the up-down direction. That is because the left-right direction was mapped onto an axis of the auditory cortex that in normal animals represents different sound frequencies and thus gets inputs from the inner ear that are precisely arranged in order of frequency. But the up-down direction was mapped onto the perpendicular axis of the auditory cortex, which ordinarily gets a mass of inputs of the same frequency. Sur also notes that the connections between the primary auditory cortex and other brain areas for hearing (the equivalent of the wiring diagram for the visual system on page 88) were unchanged by the new input.
So patterns in the input can tune a patch of sensory cortex to mesh with that input, but only within the limits of the wiring already present. Sur suggests that the reason the auditory cortex in the rewired ferrets can process visual information at all is that certain kinds of signal processing may be useful to perform on raw sensory input, whether it is visual, auditory, or tactile:
On this view, one function of sensory thalamus or cortex is to perform certain stereotypical operations on input regardless of modality [vision, hearing, or touch]; the specific type of sensory input of course provides the substrate information that is transmitted and processed. . . . If the normal organization of central auditory structures is not altered, or at least not altered significantly, by visual input, then we might expect some operations similar to those we observe on visual inputs in operated ferrets to be carried out as well in the auditory pathway in normal ferrets. In other words, the animals with visual inputs induced into the auditory pathway provide a different window on some of the same operations that should occur normally in auditory thalamus and cortex. 61
The suggestion that the auditory cortex is inherently suited to analyze visual input is not far-fetched. I mentioned that frequency (pitch) in hearing behaves a lot like space in vision. The mind treats soundmakers with different pitches as if they were objects at different locations, and it treats jumps in pitch like motions in space. 62 This means that some of the analyses performed on sights may be the same as the analyses performed on sounds, and could be computed, at
? ? ? ? ? ? ? ? ? ? least in part, by similar kinds of circuitry. Inputs from an ear represent different frequencies; inputs from an eye represent spots at different locations. Neurons in the sensory cortex (both visual and auditory) receive information from a neighborhood of input fibers and extract simple patterns from them. Therefore neurons in the auditory cortex that ordinarily detect {97} rising or falling glides, rich or pure tones, and sounds that come from specific places may, in the rewired ferrets, automatically be capable of detecting lines of specific slants, places, and directions of movement.
This is not to say that the primary auditory cortex can handle visual input right out of the box. The cortex still must tune its synaptic connections in response to the patterns in the input. The rewired ferrets are a remarkable demonstration of how the developing sensory cortex organizes itself into a well-functioning system. But as in the other examples of plasticity, they do not show that input from the senses can transform an amorphous brain into doing whatever would come in handy. The cortex has an intrinsic structure that allows it to perform certain kinds of computation. Many examples of "plasticity" may consist o~f making the input mesh with that structure.
Anyone who has watched the Discovery Channel has seen footage of baby wildebeests or zebras falling out of the birth canal, wobbling on shaky legs for a minute or two, and then prancing around their mothers with their senses, drives, and motor control fully operational. It happens far too quickly for patterned experience to have organized their brains, so there must be genetic mechanisms capable of shaping the brain before birth. Neuroscientists were aware of this before plasticity came into vogue. The first studies of the development of the visual system by David Hubel and Torsten Wiesel showed that the microcircuitry of monkeys is pretty much complete at birth. 63 Even their famous demonstrations that the visual systems of cats can be altered by experience during a critical period of development (by being reared in the dark, in striped cylinders, or with one eye sewn shut) show only that experience is necessary to maintain the visual system and to retune it as the animal grows. They do not show that experience is necessary to wire up the brain to start with.
We know in a general way how the brain assembles itself under the guidance of the genes. 64 Even before the cortex has been formed, the neurons destined to make up different areas are organized into a"proto-map. " Each area in the proto-map is composed of neurons with different properties, molecular mechanisms that attract different input fibers, and different patterns of responses to the input. Axons are attracted and repelled by many kinds of molecules dissolved in the surrounding fluid or attached to the membranes of neighboring cells. And different sets of genes are expressed in different parts of the growing cortex. The neuroscientist Lawrence Katz has lamented that fire-together- wire-together has become a "dogma" keeping neuroscientists from exploring the full reach of these genetic mechanisms. 65
But the tide is beginning to turn, and recent discoveries are showing how parts of the brain can organize themselves without any information from the senses. In experiments that the journal Science called "heretical," Katz's team removed one or both eyes from a developing ferret, depriving the visual cortex {98} of all its input. Nonetheless, the visual cortex developed with the standard arrangement of connections from the two eyes. 66
Genetically engineered mice have provided especially important clues, because knocking out a single gene can be more precise than the conventional techniques of poisoning neurons or slicing up the brain. One team invented a mouse whose synapses were completely shut down, preventing neurons from signaling to one another. Its brain developed fairly normally, complete with layered structures, fiber pathways, and synapses in the right places. 67 (The brain degenerated quickly after birth, showing again that neural activity may be more important in maintaining the brain than in wiring it. ) Another team designed a mouse with a useless thalamus, depriving the entire cortex of its input. But the cortex differentiated into the normal layers and regions, each with a different set of turned-on genes. 68 A third study did the opposite, inventing mice that were missing one of the genes that lay down gradients of molecules that help organize the brain by triggering other genes in particular places. The missing gene made a big difference: the boundaries among cortical areas were badly warped. 69 The studies with knockout mice, then, suggest that genes may be more important than neural activity in organizing the cortex. Neural activity undoubtedly plays a role, which depends on the species, the stage of development, and the part of the brain, but it is just one capability of the brain rather than the source of its structure.
What about our own species? Recall that a recent study of twins showed that differences in the anatomy of the cortex, particularly the amount of gray matter in different cortical regions, are under genetic control, paralleling differences in intelligence and other psychological traits. 70 And demonstrations of the plasticity of the human brain do not rule out substantial genetic organization. One of the most commonly cited examples of plasticity in both humans and monkeys is that the cortex dedicated to an amputated or numbed body part may get reallocated to some other body part. But the fact that the input can change the brain once it is built does not mean that the input molded the brain in the first place. Most amputees experience phantom limbs: vivid, detailed hallucinations of the missing body part.
? ? ? ? ? ? ? ? ? ? Amazingly, a substantial proportion of people who were born with a limb missing experience these apparitions as well. 71 They can describe the anatomy of their phantom limb (for example, how many toes they feel in a nonexistent
foot) and may even feel that they are gesturing with their phantom hands during conversation. One girl solved arithmetic problems by counting on her phantom fingers! The psychologist Ronald Melzack, who documented many of these cases, proposed that the brain contains an innate "neuromatrix," distributed across several cortical and subcortical regions, dedicated to representing the body.
The impression that human brains are limitlessly plastic has also come from demonstrations that children can sometimes recover from early brain {99} damage. But the existence of cerebral palsy -- lifelong difficulties with motor control and speech caused by malformations or early damage in the brain -- shows that even the plasticity of a child's brain has severe limits. The most famous evidence for extreme plasticity in humans had been the ability of some children to grow up relatively normal even with an entire hemisphere surgically removed in infancy. 72 But that may be a special case, which arises from the fact that the primate brain is fundamentally a symmetrical organ. The typically human asymmetries -- language more on the left, spatial attention and some emotions more on the right -- are superimposed on that mostly symmetrical design. It would not be surprising if the hemispheres were genetically programmed with pretty much the same abilities, together with small biases that lead each hemisphere to specialize in some talents while letting others wither. With one hemisphere gone, the remaining one has to put all its capabilities to full use.
What happens when a child loses a part of the cortex in both hemispheres, so neither hemisphere can take over the job of the missing part in the other? If cortical regions are interchangeable, plastic, and organized by the input, then an intact part of the brain should take over the function of the missing parts. The child may be a bit slower because he is working with less brain tissue, but he should develop a full complement of human faculties. But that is not what seems to happen. Several decades ago, neurologists studied a boy who suffered a temporary loss of oxygen to the brain and lost both the standard language areas in the left hemisphere and their mirror images on the right. Though he was just ten days old when he sustained the damage, he grew into a child with permanent difficulties in speaking and understanding. 73
That case study, like many in pediatric neurology, is not scientifically pure, but recent studies on two other mental faculties echo the point that babies' brains may be less plastic than many people think. The psychologist Martha Farah and her collaborators recently reported the case of a sixteen-year-old boy who contracted meningitis when he was one day old and suffered damage to the visual cortex and to the bottom of the temporal lobes on both sides of his brain. 74 When adults sustain such damage, they lose the ability to recognize faces and also have some trouble recognizing animals, though they often can recognize words, tools, furniture, and other shapes. The boy had exactly this syndrome. Though he grew up with normal verbal intelligence, he was utterly incapable of recognizing faces. He could not even recognize pictures of the cast of his favorite television show, Baywatch, which he had seen for an hour a day for the preceding year and a half. Without the appropriate strips of brain, sixteen years of seeing faces and plenty of available cortex were not enough to give him the basic human ability to recognize other people by sight. The neuroscientists Steven Anderson, Hannah and Antonio Damasio, and their colleagues recently tested two young adults who had sustained damage to {100} their ventromedial and orbital prefrontal cortex when they were young children. 75 These are the parts of the brain that sit above the eyes and are important for empathy, social skills, and self-management (as we know from Phineas Gage, the railroad worker whose brain was impaled by a tamping iron). Both children recovered from their injuries and grew up with average IQs in stable homes with normal siblings and college-educated parents. If the brain were really homogeneous and plastic, the healthy parts should have been shaped by the normal social environment and taken oyer the functions of the damaged parts. But that is not what happened with either of the children. One, who had been run over by a car when she was fifteen months old, grew into an intractable child who ignored punishment and lied compulsively. As a teenager she shoplifted, stole from her parents, failed to win friends, showed no empathy or remorse, and was dangerously uninterested in her own baby. The other patient was a young man who had lost similar parts of his brain to a tumor when he was three months old. He too grew up friendless, shiftless, thieving, and hotheaded. Along with their bad behavior, both had trouble thinking through simple moral problems, despite having IQs in the normal range. They could not, for example, say what two people should do if they disagreed on which TV channel to watch, or decide whether a man ought to steal a drug to save his dying wife.
These cases do more than refute the doctrine of extreme plasticity. They set a challenge for the genetics and neuroscience of the twenty-first century. How does the genome tell a developing brain to differentiate into neural networks that are prepared for such abstract computational problems as recognizing a face or thinking about the interests of other people? ~
? ? ? ? ? ? ? The Blank Slate has made its last stand, but, as we have seen, its latest scientific fortifications are illusory. The human genome may have a smaller number of genes than biologists had previously estimated, but that only shows that the number of genes in a genome has little to do with the complexity of the organism. Connectionist networks may explain some of the building blocks of cognition, but they are too underpowered to account for thought and language on their own; they must be innately engineered and assembled for the tasks. Neural plasticity is not a magical protean power of the brain but a set of tools that help turn megabytes of genome into terabytes of brain, that make sensory cortex dovetail with its input, and that implement the process called learning.
Therefore genomics, neural networks, and neural plasticity fit into the picture that has emerged in recent decades of a complex human nature. It is not, of course, a nature that is rigidly programmed, impervious to the input, free of culture, or endowed with the minutiae of every concept and feeling. But it is a nature that is rich enough to take on the demands of seeing, moving, {101} planning, talking, staying alive, making sense of the environment, and negotiating the world of other people.
The aftermath of the Blank Slate's last stand is a good time to take stock of the case for the alternative. Here is my summary of the evidence for a complex human nature, some of it reiterating arguments from previous chapters, some of it anticipating arguments in chapters to come.
Simple logic says there can be no learning without innate mechanisms to do the learning. Those mechanisms must be powerful enough to account for all the kinds of learning that humans accomplish. Learnability theory -- the mathematical analysis of how learning can work in principle -- tells us there are always an infinite number of generalizations that a learner can draw from a finite set of inputs. 76 The sentences heard by a child, for example, can be grounds for repeating them back verbatim, producing any combination of words with the same proportion of nouns to verbs, or analyzing the underlying grammar and producing sentences that conform to it. The sight of someone washing dishes can, with equal logical justification, prompt a learner to try to get dishes clean or to let warm water run over his fingers. A successful learner, then, must be constrained to draw some conclusions from the input and not others. Artificial intelligence reinforces this point. Computers and robots programmed to do humanlike feats are invariably endowed with many complex modules. 77
Evolutionary biology has shown that complex adaptations are ubiquitous in the living world, and that natural selection is capable of evolving them, including complex cognitive and behavioral adaptations. 78 The study of the behavior of animals in their natural habitat shows that species differ innately from one another in their drives and abilities, some of them (like celestial navigation and food caching) requiring complicated and specialized neural systems. 79 The study of humans from an evolutionary perspective has shown that many psychological faculties (such as our hunger for fatty food, for social status, and for risky sexual liaisons) are better adapted to the evolutionary demands of our ancestral environment than to the actual demands of the current environment. 80 Anthropological surveys have shown that hundreds of universals, pertaining to every aspect of experience, cut across the world's cultures. 81
Cognitive scientists have discovered that distinct kinds of representations and processes are used in different domains of knowledge, such as words and rules for language, the concept of an enduring object for understanding the physical world, and a theory of mind for understanding other people. 82 Developmental psychology has shown that these distinct modes of interpreting experience come on line early in life: infants have a basic grasp of objects, numbers, faces, tools, language, and other domains of human cognition. 83
The human genome contains an enormous amount of information, both in the genes and in the noncoding regions, to guide the construction of a {102} complex organism. In a growing number of cases, particular genes can be tied to aspects of cognition, language, and personality. 84 When psychological traits vary, much of the variation comes from differences in genes: identical twins are more similar than fraternal twins, and biological siblings are more similar than adoptive siblings, whether reared together or apart. 85 A person's temperament and personality emerge early in life and remain fairly constant throughout the lifespan. 86 And both personality and intelligence show few or no effects of children's particular home environments within their culture: children reared in the same family are similar mainly because of their shared genes. 87
Finally, neuroscience is showing that the brain's basic architecture develops under genetic control. The importance of learning and plasticity notwithstanding, brain systems show signs of innate specialization and cannot arbitrarily substitute for one another. 88
In these three chapters I have given you a summary of the current scientific case for a complex human nature. The rest of the book is about its implications.
? ? ? ? ? ? ? ? ? ?
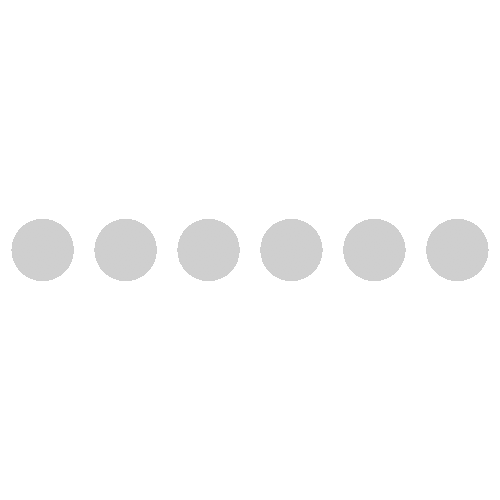